Complex coacervation drives superior core-shell fiber moisture electric generators
Keywords
Moisture electric generators (MEGs) are an emerging green energy harvesting technology that generates electricity through direct interactions between nanomaterials/structures and moisture[1-4]. As a parallel development alongside photovoltaics, triboelectric generators, and thermoelectric generators, MEGs stand out due to their independence from time and spatial constraints[5-7]. They harness ubiquitous moisture in the environment as an energy transmission medium, embodying the principle: “Where there is moisture, there is electricity”. The most extensively studied and representative MEG is the sandwich-structured membrane-based device, in which the active material is positioned in the middle layer, flanked by two electrodes on the top and bottom. Typically, the top electrode is either perforated or partially covers the active layer, while the bottom electrode fully seals it. Upon exposure to moisture, a difference in water permeability between the two electrodes results in asymmetric ionization of the active material, generating unequal amounts of mobile ions at both ends. This asymmetry establishes an ion concentration gradient, which drives the diffusion of charge carriers along the gradient and consequently induces an ionic current, giving rise to an electrical output in the external circuit[8-10]. Since the concept was introduced in 2015, various material compositions, device architectures, and macroscopical structures have been developed for MEGs[3,8]. However, the limited ion diffusion efficiency of these devices has constrained their performance, while the use of rigid metal electrodes has resulted in poor flexibility, posing challenges for applications in flexible electronics and wearable devices[5,11,12].
To address these issues, Zan et al. recently developed a high-performance, ultra-flexible fiber-based MEG device[13]. This device uses a sodium alginate/poly(diallyldimethylammonium chloride) (NaAlg/PDDA) hydrogel shell, formed via a complex coacervation strategy, as the active material coated on a poly(3,4-ethylenedioxythiophene) (PEDOT) fiber core [Figure 1A][5]. An ultra-thin copper wire serves as the external electrode, encapsulating the fiber to form the MEG device. This approach not only introduces a new method for synthesizing polymer fibers but also significantly enhances MEG performance, achieving one of the highest reported values to date [Figure 1B]. Furthermore, the material’s flexibility enables its integration into novel human-machine interaction scenarios.
Figure 1. (A) Schematic illustration of the fiber-structured MEG and its performance enhancement mechanism; (B) Power output comparison between the fiber-based MEG and other reported MEGs; (C) High-resolution C 1 s XPS spectra of PEDOT-core-based fibers and the PDDA film; (D and E) Schematic representation of the KPFM measurement setup for the PEDOT@PDDA/NaAlg fiber and corresponding KPFM images of the two regions highlighted in (D); (F and G) Molecular configurations of pristine NaAlg, pristine PDDA, and the assembled PDDA/NaAlg complex, where blue chains depict NaAlg and cyan chains represent PDDA; (H and I) Optimized equilibrium structures of PDDA/NaAlg complex (H) and pure NaAlg (I); (J) Schematic illustration of a biological synapse triggered by an action potential and an artificial synaptic device powered by the MEG potential. MEG: Moisture electric generators; XPS: X-ray photoelectron spectroscopy; PEDOT: poly(3,4-ethylenedioxythiophene); PDDA: poly(diallyldimethylammonium chloride); NaAlg: sodium alginate; KPFM: Kelvin probe force microscopy.
In this study, complex coacervation plays a central role in the fabrication of the core-shell fibers. The concept of complex coacervation dates back nearly a century. It refers to the liquid-liquid phase separation driven by electrostatic interactions between oppositely charged polymers, leading to the formation of a dense, polymer-rich coacervate phase[14]. Consequently, the network formation in complex coacervates relies on physical interactions rather than covalent bonds. The components involved in the coacervation process can include a wide variety of charged species - such as colloids, proteins, surfactants, or polymers. This method is characterized by its simplicity and rapid fabrication: mixing two components under suitable pH and ionic conditions can trigger instantaneous gelation, without requiring specialized equipment such as ultraviolet (UV) lamps or thermal ovens. This technique has broad applications in pharmaceuticals, food, cosmetics, and water treatment. Here, PEDOT fibers obtained via wet-spinning were sequentially immersed in optimized concentrations of CaCl2 solution and NaAlg/PDDA mixed solution. This process facilitated strong electrostatic interactions between the positively charged PDDA and negatively charged NaAlg backbones, forming a robust coacervate gel that tightly adhered to the PEDOT surface, creating PEDOT@NaAlg/PDDA core-shell fibers. The fabrication method is simple, rapid, and conducive to large-scale production. Characterization techniques such as Fourier transform infrared (FTIR) and X-ray photoelectron spectroscopy (XPS) revealed characteristic peak shifts in the NaAlg/PDDA coacervate compared to pure NaAlg and PDDA, verifying the presence of strong electrostatic attractions between the carboxyl groups of NaAlg and the quaternary ammonium moieties in PDDA, indicative of successful complex coacervation [Figure 1C].
Moreover, complex coacervation significantly enhances MEG performance. Comparative studies demonstrated that the MEG performance of the NaAlg/PDDA coacervate far exceeded that of pure NaAlg or PDDA. Under conditions of 20% RH and 25 °C, a pure NaAlg-based MEG only outputs an open-circuit voltage (Voc) of 300 mV and a volumetric short-circuit current density (isc) of 5 mA·cm3, while a pure PDDA-based MEG generated a Voc of 200 mV and a lower current density. In contrast, the optimized NaAlg/PDDA coacervate-based MEG delivered a high Voc of 750 mV and an exceptional maximum isc of
Complex coacervation was subsequently investigated in detail to elucidate its mechanism for enhancing the performance of MEG. Results revealed that ion dissociation and their uneven distribution along the radial direction within the NaAlg/PDDA coacervate fiber were the primary contributors to electricity generation [Figure 1D and E]. Zeta potential measurements indicated that the NaAlg/PDDA coacervate exhibited a higher absolute zeta potential compared to the individual components, enhancing its charge separation capability. Small-angle X-ray scattering analysis revealed a contracted polymer framework and increased branched structures within the NaAlg/PDDA coacervate, creating more ion diffusion pathways and facilitating charge carrier transport. Additionally, X-ray diffraction (XRD) results identified NaCl crystalline peaks in the NaAlg/PDDA coacervate, absent in pure NaAlg, suggesting the release of free ions (Na+ and Cl-) that improved MEG performance following moisture absorption. Conductivity tests further confirmed that the ionic conductivity of the NaAlg/PDDA coacervate was ten times that of pure NaAlg. These findings demonstrate that complex coacervation induces polymer framework contraction, generating more free volume and mobile charge carriers, thereby significantly improving ion diffusion efficiency. The proposed theory was further validated through Density Functional Theory calculations [Figure 1F-I].
The NaAlg/PDDA coacervate, prepared via complex coacervation, also exhibits hydrogel-like flexibility. Combined with the highly conductive and flexible PEDOT fiber core that is capable of withstanding over 100,000 bending cycles without damage, this design overcomes the rigidity of metal electrodes. This study has revealed that the intrinsic negative surface potential of PEDOT can establish a built-in potential, which effectively accelerates carrier diffusion and enhances power generation performance. The exceptional flexibility of this device, combined with its high energy output, significantly broadens its potential application scenarios. The device is well-suited for self-powered information exchange. A fiber-based MEG (moisture-enabled generator) was designed to be worn on the finger, enabling the generation of dot- and dash-like current signals by modulating the duration of finger bending. This mimics Morse code, thereby facilitating information transmission. Furthermore, the MEG was integrated with an artificial synaptic device to construct the first self-powered artificial synapse, which exhibited promising neuromorphic plasticity. These demonstrations highlight the device’s potential in next-generation human-machine interfaces and neuromorphic computing applications [Figure 1J].
In summary, this study pioneers the application of the complex coacervation strategy in the MEG field, providing a novel approach to fabricating fiber-structured MEG materials. The contraction of oppositely charged polymer frameworks during complex coacervation, accompanied by increased free volume and mobile charge carriers, significantly enhances MEG performance. More importantly, the integration of fiber-based MEGs with artificial synapses lays the groundwork for future neuromorphic computing applications, demonstrating vast potential for development. However, this study still lacks investigations into large-scale device integration and practical validation of wearability - both of which are critical steps toward real-world application. Looking ahead, complex coacervation, with its dual advantages in synthetic feasibility and functional performance, holds significant promise for advancing self-powered flexible and wearable electronics.
DECLARATIONS
Authors’ contributions
Wrote the draft manuscript: Li, S.; Zhao, K.
Revised and rewrote some parts of the manuscript: Zan, G.
Availability of data and materials
Not applicable.
Financial support and sponsorship
The study was supported by the National Natural Science Foundation of China (No. 22205165).
Conflicts of interest
All authors declared that there are no conflicts of interest.
Ethical approval and consent to participate
Not applicable.
Consent for publication
Not applicable.
Copyright
© The Author(s) 2025.
REFERENCES
1. Liu, X.; Gao, H.; Ward, J. E.; et al. Power generation from ambient humidity using protein nanowires. Nature 2020, 578, 550-4.
2. Zhang, Z.; Li, X.; Yin, J.; et al. Emerging hydrovoltaic technology. Nat. Nanotechnol. 2018, 13, 1109-19.
3. Liu, Z.; Liu, C.; Chen, Z.; et al. Recent advances in two-dimensional materials for hydrovoltaic energy technology. Exploration 2023, 3, 20220061.
4. Zan, G.; Li, S.; Zhao, K.; et al. Emerging bioinspired hydrovoltaic electricity generators. Energy. Environ. Sci. 2025, 18, 53-96.
5. Deng, Y.; Shi, X.; Wu, T.; et al. Optimization of Wet-Spun PEDOT:PSS fibers for thermoelectric applications through innovative triple post-treatments. Adv. Fiber. Mater. 2024, 6, 1616-28.
6. Zhang, X.; Xu, J.; Zhang, X.; et al. Simultaneous evaporation and foaming for batch coaxial extrusion of liquid metal/polydimethylsiloxane porous fibrous TENG. Adv. Fiber. Mater. 2023, 5, 1949-62.
7. Zhou, B.; Shang, C.; Wang, C.; et al. Strain engineering and halogen compensation of buried interface in polycrystalline halide perovskites. Research 2024, 7, 0309.
8. Zhao, F.; Cheng, H.; Zhang, Z.; Jiang, L.; Qu, L. Direct power generation from a graphene oxide film under moisture. Adv. Mater. 2015, 27, 4351-7.
9. Zhang, S.; Fu, J.; Xing, G.; Zhu, W.; Ben, T. Recent advances in porous adsorbent assisted atmospheric water harvesting: a review of adsorbent materials. Chem. Synth. 2023, 3, 10.
10. Ren, G.; Hu, Q.; Ye, J.; Hu, A.; Lü, J.; Zhou, S. All-biobased hydrovoltaic-photovoltaic electricity generators for all-weather energy harvesting. Research 2022, 2022, 9873203.
11. Dang, C.; Liu, M.; Lin, Z.; Yan, W. Selenium nanomaterials enabled flexible and wearable electronics. Chem. Synth. 2023, 3, 14.
12. Wang, H.; Yan, W.; Zhu, M. Revolutionizing fiber batteries with polymer gel electrolyte: a groundbreaking innovation in wearable energy. Chem. Synth. 2024, 4, 57.
13. Zan, G.; Jiang, W.; Kim, H.; et al. A core-shell fiber moisture-driven electric generator enabled by synergetic complex coacervation and built-in potential. Nat. Commun. 2024, 15, 10056.
Cite This Article

How to Cite
Download Citation
Export Citation File:
Type of Import
Tips on Downloading Citation
Citation Manager File Format
Type of Import
Direct Import: When the Direct Import option is selected (the default state), a dialogue box will give you the option to Save or Open the downloaded citation data. Choosing Open will either launch your citation manager or give you a choice of applications with which to use the metadata. The Save option saves the file locally for later use.
Indirect Import: When the Indirect Import option is selected, the metadata is displayed and may be copied and pasted as needed.
About This Article
Copyright
Data & Comments
Data
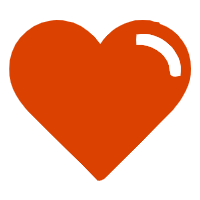
Comments
Comments must be written in English. Spam, offensive content, impersonation, and private information will not be permitted. If any comment is reported and identified as inappropriate content by OAE staff, the comment will be removed without notice. If you have any queries or need any help, please contact us at [email protected].