Circularly polarized luminescence in chiral nematic liquid crystals based on photochromic molecules
Abstract
Circularly polarized luminescence (CPL) has emerged as a focal point in luminescent materials research, reflecting a growing field of study. Responding to the demand for practical applications across diverse sectors, there is a critical need for CPL materials with high luminescence dissymmetry factors (glum) and adjustable optical performance, driving the development of novel materials and methodological approaches. Chiral nematic liquid crystals (N*-LCs) have attracted significant attention as promising candidates for producing high-performance CPL materials due to their ability to exhibit enhanced glum and modulate circularly polarized light. This review comprehensively presents the stimuli-responsive CPL properties, utilizing N*-LCs as chiral templates doped with various photochromic compounds, particularly azobenzene, spiropyran (SP), diarylethene and overcrowded alkene. By elucidating the dynamic interplay between molecular structure, supramolecular organization, and optical response, it offers a profound understanding of the stimuli-responsive CPL behaviors. Additionally, the review also explores the multifaceted applications of photochromic N*-LCs materials, spanning advanced display technologies, optical data storage, and beyond. By examining diverse avenues for leveraging their unique optical properties, it highlights their pivotal role in driving innovation across photonic applications, facilitating transformative advancements in the field.
Keywords
INTRODUCTION
Circularly polarized luminescence (CPL) has emerged as a compelling area of research within the domain of luminescent materials, driven by its promising applications in diverse fields including photonics, optoelectronics, and biomedicine[1-11]. The realization of these applications hinges upon the development of CPL-active materials possessing both high luminescence dissymmetry factors (glum), which quantify the degree of circular polarization[12] in emitted light, and tunable optical properties tailored to specific requirements[13]. In terms of biomedicine, CPL materials hold promise for applications such as bioimaging and diagnostics. The ability to selectively emit circularly polarized light enables enhanced contrast and sensitivity in imaging techniques, facilitating the visualization and analysis of biological samples with high precision and accuracy. In recent years, significant endeavors have been made in the design and development of functional CPL materials, with a focus on enhancing their optical performance and versatility for tailored applications. To date, numerous emerging CPL-active materials have been reported, encompassing metal complexes[14-19], organic and inorganic small molecules[20-24], synthetic polymers[25-27], self-assembly systems[28-32], and chiral nematic liquid crystals (N*-LCs)[33-35]. However, the glum values of most chiral luminescent materials are relatively low. In the realm of practical applications, the necessity of developing appropriate CPL materials with a significant degree of polarization has become increasingly pressing. Among these, N*-LCs have emerged as promising candidates owing to their inherent chirality and facile tunability, offering a versatile platform for bestowing circular polarization upon luminescent systems. This capability facilitates precise control over the polarization state of emitted light.
Functional liquid crystal materials exhibiting CPL properties have undergone extensive investigation across various applications. In this context, chirality confers unprecedented optical, photonic, and mechanical attributes to these distinctive soft materials. N*-LCs serve as quasi-one-dimensional photonic crystals and can be directly obtained from cholesterol derivatives or by blending chiral dopants into nematic liquid crystals[36]. This foundational aspect sets the stage for exploring the development of CPL materials based on N*-LCs. In the fabrication of circularly polarized luminescent materials, a common approach involves introducing either chiral or achiral luminescent materials into achiral or chiral liquid crystals, respectively. This strategy enables the modulation of the CPL properties tailored to the specific application requirements[37-39]. The utilization of diverse luminescent materials such as luminogens with aggregation-induced emission (AIE) properties, organic fluorescence molecules, quantum rods, inorganic perovskite nanocrystals, carbon dots, semiconductor quantum nanocrystals, conjugated polymers and upconversion nanoparticles enriches the circularly polarized active materials based on N*-LCs. This diversity contributes to the versatility and tunability of the constructed systems. Recent advancements in the field have led to the development of stimuli-responsive CPL materials based on N*-LCs, which have attracted considerable attention. Various external stimuli, including light, pH, solvents, electric fields, temperature and force, have been employed to adjust CPL properties in N*-LCs media. These stimuli offer opportunities for dynamic control and manipulation of CPL signals in the constructed systems. From a mechanistic perspective, CPL switching in emissive N*-LCs systems can be achieved by adjusting molecular structures to alter the related helical assembly behavior or by regulating external interactions between achiral and chiral components. This mechanistic understanding enhances the design and optimization of CPL-active materials based on
This review aims to comprehensively investigate the current landscape of CPL materials, with a specific focus on CPL based on N*-LCs doped with various photochromic compounds [Figure 1]. By examining the unique characteristics of CPL in N*-LCs-hosted systems containing a variety of photochromic molecules such as azobenzene, spiropyran (SP), diarylethene and overcrowded alkene, the potential mechanisms and strategies for enhancing CPL performance are elucidated. Additionally, the effective control of stimuli-responsive N*-LCs circularly polarized luminescent materials based on photochromic compounds is discussed, emphasizing the potential for dynamic control over CPL properties and thereby expanding the application scope of CPL in responsive and adaptive optoelectronic devices. Finally, this paper provides a forward-looking perspective on the potential opportunities and challenges inherent in stimuli-responsive CPL-active materials based on N*-LCs architectures, aiming to guide future research efforts toward the rational design and engineering of CPL materials tailored for specific applications. It is anticipated that this comprehensive review will serve as a valuable resource for researchers and practitioners in the field, driving further advancements in CPL materials and technologies.
CPL
The CPL property refers to the emission of light from a system after excitation by non-circularly polarized light, containing a higher proportion of left- or right-handed components. When two beams of light are combined, they exhibit elliptical polarization characteristics. As illustrated in Figure 2, for non-chiral molecules or assemblies in a homogeneous solution, there is no preference for left or right circular polarization in the emitted light when returning to the ground state via radiative transitions, i.e., IL = IR, where IR and IL represent the intensities of right-handed and left-handed circularly polarized light in the emitted luminescence, respectively. However, for chiral systems, when chiral molecules or assemblies emit light upon returning to the ground state after excitation, the proportion of emitted left-handed or right-handed circularly polarized light is higher, i.e., IL ≠ IR, thus demonstrating the property of CPL.
Figure 2. The schematic diagram illustrating the principle of CPL testing for (A) non-chiral systems, and (B) for chiral systems. CPL: Circularly polarized luminescence.
According to the above formula, the dissymmetry factor of CPL glum ranges between -2 and +2. When glum = +2, it indicates that the system emits only left-handed circularly polarized light. Conversely, when glum = -2, it signifies that the system emits only right-handed circularly polarized light. A value of glum = 0 indicates that the emitted light from the system is unpolarized.
N*-LCS
N*-LCs molecules exhibit a multi-layered arrangement, with rod-shaped liquid crystal molecules oriented unidirectionally within each layer, while the orientations between layers form a certain angle, resulting in an overall helical structure of the liquid crystal molecules[37,40-44]. N*-LCs demonstrate various optical properties:
(1) Selective reflection
The selective reflection of N*-LCs manifests when white light irradiates the surface of N*-LCs with a specific pitch. N*-LCs selectively reflect light of a certain wavelength. If the reflected light falls within the visible light range of 400-780 nm, it can be perceived by the naked eye. This refined characterization is essential for understanding the structural nuances of these complex materials. In the realm of N*-LCs, handedness and helical pitch (p) are paramount parameters for delineating the molecular helical arrangement. Handedness, denoted as left- or right-handedness, articulates the direction of molecular rotation around the helical axis. The helical pitch is delineated as the axial distance required for the director to complete a full 360°. According to Bragg’s law, the selective reflection characteristic of N*-LCs, organized into helical layered structures, follows the Bragg equation, where the central wavelength (λ) of the selectively reflected band is proportional to the helical pitch and the average refractive index (n) of N*-LCs, expressed as λ = np. By adjusting the pitch length through external fields (light, electric, thermal), N*-LCs reflect light of different colors, facilitating their widespread application in various color display technologies and specialty reflective film technologies.
(2) Optical activity
When N*-LCs liquid crystals are placed between two polarizers oriented at 90° to each other, light can still effectively transmit through. However, depending on the arrangement of liquid crystal molecules, the brightness of the transmitted light varies according to the molecular alignment state. Hence, polarizing microscopy can be employed to observe the phase and texture of N*-LCs.
(3) Circular dichroism of circularly polarized light
Natural light can be divided into right-handed circularly polarized light and left-handed circularly polarized light. Only circularly polarized light whose rotation matches the helical structure of N*-LCs is reflected, while the remaining light is absorbed or transmitted[35]. This phenomenon is termed the circular dichroism property of N*-LCs.
STIMULI-RESPONSIVE CPL IN N*-LCS
Azobenzene derivatives
Azobenzene is a compound with unique photoresponsiveness, primarily attributed to its azo group
A multi-component co-assembly approach was employed to generate extensive spiral spherulites featuring chiroptical switching within an achiral liquid crystal block copolymer (LC-BCP) framework [Figure 3A]. Yuan et al. prepared controllable chiral spherulite films by co-assembling achiral LC-BCP, chiral tartaric acid (TA) molecules, and fluorescent molecules [Rhodamine B (RhB)] at the liquid crystalline temperature. Chiral transfer between RhB, TA and LC-BCP was facilitated through non-covalent interactions, as confirmed by chiral spectroscopy[46]. Electron and optical microscopy confirmed the macroscopic chiral hierarchical structures. The fluorescent molecules adopted a helical arrangement induced by the chiral template, resulting in orange CPL emission under 530 nm excitation, with a dissymmetry factor of up to 0.02. Additionally, the film’s fluorescence could be erased under UV excitation via azobenzene cis-trans isomerization and recovered after thermal annealing [Figure 3B]. This methodology presents a streamlined avenue for manufacturing expansive chiral spherulites, presenting a promising tactic for fabricating chiroptical materials. Kang et al. proposed an innovative molecular design strategy aimed at independently regulating photoisomerization and fluorescence architectures[47]. They developed azoarene-based CPL molecular switches, namely (S, R, S)-switch 1 and control (R, R, R)-switch 2, incorporating the (R)-binaphthyl azo group as the chiral photosensitive moiety and (S) or (R)-binaphthyl-linked fluorescent molecules as chiral fluorescent moieties. Both switches demonstrated reversible trans/cis photoisomerization upon exposure to UV light at 365 nm and green light at 520 nm, in both solvent and liquid crystal environments [Figure 3C]. In the resulting chiral liquid crystal induced by switch 1, customizable CPL behaviors were attained, encompassing polarization inversion and switching-off, while upholding comparable fluorescence intensity under both light wavelengths. The investigation underscored a proof-of-concept for a “CPL optical Morse code” utilizing chiral liquid crystals containing switch 1, underscoring the potential of optically- and thermally-responsive CPL active materials facilitated by chiral molecular photo-switches for stimuli-controllable chiroptical devices [Figure 3D].
Figure 3. (A) Macroscopic chiral spherulites (≈ 1 mm) formed by co-assembling LC-BCP, RhB, and chiral TA, illustrating their evolution; (B) The CPL and glum spectra of annealed films LC-BCP/L-TA/RhB and LC-BCP/D-TA/RhB were analyzed, elucidating the molecular and assembly structural alterations induced by UV irradiation and subsequent thermal re-annealing. Reproduced with permission from[46]. Copyright 2023, Wiley-VCH. GmbH; (C) The chemical configurations of molecular switches (S, R, S)-switch 1 and (R, R, R)-switch 2 were depicted, illustrating their respective helical handedness and CPL signals within the CLC system upon light irradiation; (D) Message encryption and decryption employing dual-mode fluorescence and CPL. Reproduced with permission from[47]. Copyright 2023, Wiley-VCH. GmbH. LC-BCP: Liquid crystal block copolymer; RhB: Rhodamine B; TA: tartaric acid; CPL: circularly polarized luminescence; CLC: cholesteric liquid crystal.
SP derivatives
SP molecules exhibit multiple responsiveness upon external stimuli, including photoresponsiveness, thermoresponsiveness, chemical responsiveness, and mechanical responsiveness[48]. Upon irradiation with UV light, the molecule undergoes a ring-opening reaction, transitioning into a colored zwitterionic merocyanine (MC) state. Subsequent heating or irradiation with visible light reverses the MC form back to the colorless SP form. This reversible transformation, accompanied by notable changes in geometry, color and charge distribution, results in discernible variations in photophysical characteristics between the two states. Such reversible interconversion offers a plethora of design possibilities for various applications. This photoresponsiveness endows SP molecules with potential applications as optical switches, photosensitive dyes, and other optically functional materials.
Recently, Shi et al. introduced a novel multi-mode anti-counterfeiting material suitable for security labels and encryption encoding[49]. The material is composed of excess photofluorochromic SP and a small quantity of Zn2+ dispersed into nematic liquid crystals. Under UV light, the system exhibits the coexistence of MC and MC-Zn2+, with energy transfer occurring from MC-Zn2+ to MC [Figure 4A]. This results in a purple color appearance and intense red CPL emission with a high |glum| value of up to 1.1 at 685 nm. Upon heating to approximately 50 °C, MC is rapidly converted to an SP isomer, while the MC-Zn2+ isomer remains unchanged, resulting in an orange-colored SP/MC-Zn2+-N*LC system with good CPL performance and a |glum| value of up to 0.4 at 590 nm. Furthermore, under visible light irradiation, MC and MC-Zn2+ can be easily converted to a colorless SP, releasing Zn2+ (SP/Zn2+-N*LC). Compared to previous multi-mode materials, this system offers several advantages: wide material availability without requiring complex synthesis, reversible conversion of each mode by light or heat stimulation, and a high |glum| value facilitating clear discrimination of reflective and fluorescence colors in each state. These findings not only advance anti-counterfeiting technology but also pave the way for the rational design and scalable production of advanced anti-counterfeiting materials utilizing stimuli-responsive materials coupled with N*LC [Figure 4B]. Kang
Figure 4. (A) Advanced anti-counterfeiting materials with reflective color, fluorescence, and CPL signals, each having three reversible states; insets show photographs under natural and 365 nm light using glasses with R-CPF and L-CPF filters; (B) Advanced information encryption/decryption utilizing fluorescence, reflective color, and CPL, demonstrating dynamic variations. Reproduced with permission from[49]. Copyright 2022, Wiley-VCH. GmbH; (C) Hierarchical assembly for macroscopic, bio-inspired, chiral emissive CNC-SP nanostructures; (D) Chiral patterning via chemical treatment for controlling CPL handedness and chiral emission wavelength, facilitating enhanced security optical labeling through the utilization of quantum dot codes imprinted onto chiral emissive CNC-SP film via photochemical printing. Reproduced with permission from[50]. Copyright 2021, Wiley-VCH. GmbH. CPL: Circularly polarized luminescence; CPF: circular polarized filter; CNC: cellulose nanocrystal; SP: spiropyran.
Diarylethene derivatives
The process of diarylethene molecules undergoing cis-trans isomerization upon exposure to light is typically achieved through photoisomerization reactions[51]. The development of fluorescent photochromic diarylethene-based compounds includes α-cyanodiarylethenes, α-cyanostilbenes, and diaryldicyanoethenes[52]. Regarding α-cyanodiarylethene, when subjected to UV or visible light irradiation, photon energy excites the double bonds (C=C bonds), leading to the breaking and formation of new bonds. Consequently, this process often results in the formation of cis and trans isomers. In the cis isomer, the two aryl groups of the molecule are positioned on the same side, whereas in the trans isomer, these two aryl groups are positioned on opposite sides. This light-induced cis-trans isomerization induces changes in the molecular conformation and electronic distribution, thereby influencing their optical, electrical, and chemical properties. This responsiveness endows α-cyanodiarylethene molecules with broad potential applications in fields such as smart materials, optoelectronic devices, and chemical sensors.
Lin et al. had successfully developed a Förster Resonance Energy Transfer (FRET) platform within a cholesteric liquid crystal (CLC) film [Figure 5A][53]. This innovative system utilized a conventional dye, C6, as the energy donor, and a chiral photoswitch 7 based on 1,2-dithienyldicyanoethene, as the energy acceptor. The platform demonstrated efficient FRET from C6 to switch 7 in its initial state, which was attributed to the excellent spectral overlap between the emission spectrum of the donor and the absorption spectrum of the acceptor. Upon exposure to green light at 520 nm, switch 7 underwent a trans-cis photoisomerization, which effectively “turns off” the FRET process. This change was due to a reduced overlap between the absorption spectrum of the cis-isomer of switch 7 and the emission spectrum of C6, leading to a decrease in energy transfer efficiency. However, this process can be partially reversed by irradiation with 365 nm UV light. The CLC film exhibited the remarkable ability to dynamically modulate the fluorescent color between green and orange through the FRET process, which varied in efficiency due to the reversible trans-cis photoisomerization of switch 7. Additionally, preliminary experiments had showcased the electro-optic response of the CLC film, demonstrating the capability to switch among the planar, homeotropic, and focal conic states by applying an appropriate voltage across the cell. This feature underscores the potential of the CLC film for applications in information display and data encryption [Figure 5B]. Li et al. demonstrated a novel method to manipulate CPL in CLC systems[54]. By introducing a photoresponsive achiral dye (KG) and a static achiral dye (NR) with opposite dichroism, CPL handedness and intensity can be controlled [Figure 5C]. KG underwent configuration transformation under UV irradiation, weakening its negative dichroism and reducing CPL intensity. By combining NR at an appropriate ratio, CPL inversion can be achieved without changing the chiral superstructure of CLC [Figure 5D]. This dynamic behavior enables information encryption without altering the macroscopic LC structure. This work provides a new strategy for CPL modulation in CLC systems, with potential applications in multidimensional photoresponsive materials.
Figure 5. (A) Dynamic FRET systems within the helical superstructures of CLCs; (B) Potential applications in information encryption using multifunctional CLCs in PMMA microtube. Reproduced with permission from[53]. Copyright 2023, Springer Nature; (C) Schematic illustrations of CPL signal inversion driven by UV (365 nm) light without CLC superstructure change, where “L” denotes left circular polarization and “R” denotes right circular polarization; (D) The schematic diagram of information coding using the NLC-KG, CLC-KG, and CLC-KGNR-0.5 system. Reproduced with permission from[54]. Copyright 2024, Wiley-VCH. GmbH. FRET: Förster Resonance Energy Transfer; CLC: cholesteric liquid crystal; PMMA: poly(methyl methacrylate); CPL: circularly polarized luminescence; UV: ultraviolet.
Overcrowded alkene derivatives
Sterically overcrowded alkenes are composed of two halves connected via a double bond, which is forced out of planarity due to steric hindrance[55]. This deviation from planarity alleviates the steric interactions, resulting in distortion of the molecular framework and imparting intrinsic helicity to these molecules. Overcrowded alkene-based molecular motors (MM) undergo unique 360° unidirectional rotations via photo- and thermal-driven helix inversion processes, finding broad applications in responsive surfaces, photoresponsive gels, and transmembrane transportation[56]. The stimulus-controlled angular orientation movement of helical axes within self-assembled helical superstructures facilitates the dynamic reorganization of CLCs (N*-LC).
Bao et al. focused on developing high-performance CPL materials by utilizing a photoresponsive MM to trigger dynamic superstructures in N*-LC [Figure 6A][57]. By introducing alkyl chain substituents on the MM rotor to enhance compatibility, the rotary motion of the motors induced changes in the N*-LC structure, allowing for chirality and CPL intensity modulation. Co-doping four guest achiral luminescent dyes in
Figure 6. (A) Photodriven chiral inversion of CPL in dynamic cholesteric liquid crystal superstructures tuned by alternate 365 nm UV light and heat; (B) Light-dependent selective reflection bands and CPLs. Reproduced with permission from[57]. Copyright 2021, Wiley-VCH. GmbH. CPL: Circularly polarized luminescence; UV: ultraviolet.
On consideration of the above content, stimuli-responsive smart circularly polarized luminescent liquid crystal systems possess structural dynamic controllability. By introducing functional groups with stimuli responsiveness and luminescent properties into chiral liquid crystals, the intelligent responsiveness of the chiral liquid crystal assemblies is endowed. The key advantages of stimuli-responsive chiral liquid crystal systems lie in the flexibility of design and the simplicity of operation, providing more excellent options for the development of intelligent optoelectronic materials. Despite significant achievements in this field and the introduction of many outstanding studies in this review, there are still many challenges. The basic properties of functionalized molecules need to be improved to meet the requirements of practical applications. High fatigue resistance, rapid response capability, high sensitivity to stimuli, and easy operability for the transition between different states of circularly polarized light are highly desired, and the stability of each state is crucial for enhancing reproducibility and fatigue resistance. Moreover, to meet the needs of practical applications, responsive CPL systems require high photoluminescence quantum efficiency and glum. Although liquid crystal systems can achieve large glum, the maximization of glum while enhancing photoluminescence quantum efficiency necessitates the improvement of the fundamental properties of responsive CPL systems and comprehensive design and regulation of the arrangement of chiral emitters.
CONCLUSION AND OUTLOOK
In summary, the investigation into CPL utilizing N*-LCs has unveiled promising prospects for high-performance luminescent materials. Through a comprehensive synthesis of CPL characteristics and their intricate relationship with molecular structure and supramolecular organization, N*-LCs have emerged as versatile platforms for stimuli-responsive CPL materials. The incorporation of diverse photochromic compounds, including azobenzene, SP, diarylethene and overcrowded alkene, has facilitated the customization of optical responses, thereby enhancing the adaptability and versatility of CPL materials. Looking forward, further research endeavors should concentrate on exploring novel molecular designs and advanced fabrication techniques to augment the performance and scalability of CPL materials based on
Although the review identified significant challenges in advancing CPL-active materials based on N*-LCs, many challenges remain in this rapidly growing research field. On the one hand, a key among future research trajectory is a detailed exploration of the underlying mechanisms that confer high dissymmetry factors, which is pivotal for the advancement of next-generation materials. Achieving a glum significantly greater than ±1 is crucial for optimizing the performance in optoelectronic and photocatalytic applications. The enhancement of the CPL signal’s glum is influenced by various factors, including the emitter type, dopant concentration, helical pitch, and the interactions within the chiral dopants and liquid crystal matrix. Lastly, the development of innovative chiral emitters with high photoluminescence quantum yields and helical twisting power is identified as a critical need for the specific N*-LCs systems. On the other hand, the self-organized helical superstructure of N*-LCs confers sensitivity to diverse external stimuli, enabling the tunability of the CPL characteristics through alterations in the helical organization, such as helix handedness and pitch. Consequently, the development of stimulus-responsive N*-LCs-based CPL-active materials has been extensively pursued by modulating molecular structures or helical assembly behaviors. Notably, switchable CPL behaviors have been realized via various stimuli, including light, pH, solvents, temperature, and electric fields, with light emerging as a particularly advantageous external stimulus due to its non-invasive and dynamic nature. The concept of dual- or multi-stimulus-responsive N*-LCs materials has also been introduced, highlighting the potential for sophisticated material responses. However, the rapid, reversible, and real-time modulation of CPL-active N*-LCs materials remains a significant challenge for practical applications. A critical aspect of this challenge is sustaining high glum or achieving reversible control over the glum during the switching process, which is essential for the functionality of these materials in responsive systems.
In conclusion, the ongoing exploration of N*-LCs as platforms for CPL materials is poised to drive significant advancements in the field of luminescent materials and photonics, with implications spanning diverse scientific and technological domains. By harnessing the unique properties of N*-LCs and fostering interdisciplinary collaborations, we can expect transformative innovations that will revolutionize various sectors, from consumer electronics to healthcare and beyond.
DECLARATIONS
Authors’ contributions
Conceptualization, writing - original draft, validation, investigation, writing - review editing and funding acquisition: Han D
Writing - review and editing, supervision: Chen G, Peng L
Availability of data and materials
Not applicable.
Financial support and sponsorship
This work was supported by the National Natural Science Foundation of China (No. 22305216).
Conflicts of interest
All authors declared that there are no conflicts of interest.
Ethical approval and consent to participate
Not applicable.
Consent for publication
Not applicable.
Copyright
© The Author(s) 2024.
REFERENCES
1. Zhang DW, Li M, Chen CF. Recent advances in circularly polarized electroluminescence based on organic light-emitting diodes. Chem Soc Rev 2020;49:1331-43.
2. Dhbaibi K, Abella L, Meunier-Della-Gatta S, et al. Achieving high circularly polarized luminescence with push-pull helicenic systems: from rationalized design to top-emission CP-OLED applications. Chem Sci 2021;12:5522-33.
3. Sang Y, Han J, Zhao T, Duan P, Liu M. Circularly polarized luminescence in nanoassemblies: generation, amplification, and application. Adv Mater 2020;32:e1900110.
4. Han D, Yang X, Han J, Zhou J, Jiao T, Duan P. Sequentially amplified circularly polarized ultraviolet luminescence for enantioselective photopolymerization. Nat Commun 2020;11:5659.
5. Zhan X, Xu FF, Zhou Z, Yan Y, Yao J, Zhao YS. 3D laser displays based on circularly polarized lasing from cholesteric liquid crystal arrays. Adv Mater 2021;33:e2104418.
6. Chen W, Ma K, Duan P, et al. Circularly polarized luminescence of nanoassemblies via multi-dimensional chiral architecture control. Nanoscale 2020;12:19497-515.
7. Zhao T, Han J, Shi Y, Zhou J, Duan P. Multi-light-responsive upconversion-and-downshifting-based circularly polarized luminescent switches in chiral metal-organic frameworks. Adv Mater 2021;33:e2101797.
8. Jin X, Sang Y, Shi Y, et al. Optically active upconverting nanoparticles with induced circularly polarized luminescence and enantioselectively triggered photopolymerization. ACS Nano 2019;13:2804-11.
9. Li L, Li D, Dong X, et al. A confined self-assembly approach towards chiral photonic materials with circularly polarized structural colors for information storage and encryption. Chem Eng J 2024;479:147669.
10. Zhao T, Han J, Duan P, Liu M. New perspectives to trigger and modulate circularly polarized luminescence of complex and aggregated systems: energy transfer, photon upconversion, charge transfer, and organic radical. Acc Chem Res 2020;53:1279-92.
11. Liu Y, Xing P. Circularly polarized light responsive materials: design strategies and applications. Adv Mater 2023;35:e2300968.
12. Longhi G, Castiglioni E, Koshoubu J, Mazzeo G, Abbate S. Circularly polarized luminescence: a review of experimental and theoretical aspects. Chirality 2016;28:696-707.
13. He Y, Lin S, Guo J, Li Q. Circularly polarized luminescent self-organized helical superstructures: from materials and stimulus-responsiveness to applications. Aggregate 2021;2:e141.
14. Zhang C, Li S, Dong X, Zang S. Circularly polarized luminescence of agglomerate emitters. Aggregate 2021;2:e48.
15. Wu Z, Yao Q, Chai OJH, et al. Unraveling the impact of gold(I)-thiolate motifs on the aggregation-induced emission of gold nanoclusters. Angew Chem Int Ed Engl 2020;59:9934-9.
16. Jin Y, Peng QC, Xie JW, Li K, Zang SQ. Photo-activated circularly polarized luminescence film based on aggregation-induced emission copper(I) cluster-assembled materials. Angew Chem Int Ed Engl 2023;62:e202301000.
17. Wang Y, Li N, Chu L, et al. Dual enhancement of phosphorescence and circularly polarized luminescence through entropically driven self-assembly of a platinum(II) complex. Angew Chem Int Ed Engl 2024;63:e202403898.
18. Zhang J, Dai L, Webster AM, et al. Unusual magnetic field responsive circularly polarized luminescence probes with highly emissive chiral europium(III) complexes. Angew Chem Int Ed Engl 2021;60:1004-10.
19. Dong J, Liu Y, Cui Y. Supramolecular chirality in metal-organic complexes. Acc Chem Res 2021;54:194-206.
20. Zheng S, Han J, Jin X, et al. Halogen bonded chiral emitters: generation of chiral fractal architecture with amplified circularly polarized luminescence. Angew Chem Int Ed Engl 2021;60:22711-6.
21. Zhao T, Meng D, Hu Z, et al. Enhanced chiroptic properties of nanocomposites of achiral plasmonic nanoparticles decorated with chiral dye-loaded micelles. Nat Commun 2023;14:81.
22. Roose J, Tang BZ, Wong KS. Circularly-polarized luminescence (CPL) from chiral AIE molecules and macrostructures. Small 2016;12:6495-512.
23. Liu J, Yang L, Qin P, Zhang S, Yung KKL, Huang Z. Recent advances in inorganic chiral nanomaterials. Adv Mater 2021;33:e2005506.
24. Ma K, Chen W, Jiao T, et al. Boosting the circularly polarized luminescence of small organic molecules via multi-dimensional morphology control. Chem Sci 2019;10:6821-7.
25. Zhao B, Pan K, Deng J. Intense circularly polarized luminescence contributed by helical chirality of monosubstituted polyacetylenes. Macromolecules 2018;51:7104-11.
26. Zhao B, Yu H, Pan K, Tan Z, Deng J. Multifarious chiral nanoarchitectures serving as handed-selective fluorescence filters for generating full-color circularly polarized luminescence. ACS Nano 2020;14:3208-18.
27. Zhao B, Gao X, Pan K, Deng J. Chiral helical polymer/perovskite hybrid nanofibers with intense circularly polarized luminescence. ACS Nano 2021;15:7463-71.
28. Yang D, Han J, Sang Y, Zhao T, Liu M, Duan P. Steering triplet-triplet annihilation upconversion through enantioselective self-assembly in a supramolecular gel. J Am Chem Soc 2021;143:13259-65.
29. Yang D, Duan P, Zhang L, Liu M. Chirality and energy transfer amplified circularly polarized luminescence in composite nanohelix. Nat Commun 2017;8:15727.
30. Zhang G, Bao Y, Ma H, et al. Precise modulation of circularly polarized luminescence via polymer chiral co-assembly and contactless dynamic chiral communication. Angew Chem Int Ed Engl 2024;63:e202401077.
31. Gong Z, Zhu X, Zhou Z, et al. Frontiers in circularly polarized luminescence: molecular design, self-assembly, nanomaterials, and applications. Sci China Chem 2021;64:2060-104.
32. Wong H, Lo W, Yim K, Law G. Chirality and chiroptics of lanthanide molecular and supramolecular assemblies. Chem 2019;5:3058-95.
33. Yang X, Zhou M, Wang Y, Duan P. Electric-field-regulated energy transfer in chiral liquid crystals for enhancing upconverted circularly polarized luminescence through steering the photonic bandgap. Adv Mater 2020;32:e2000820.
34. Yang X, Jin X, Zheng A, Duan P. Dual band-edge enhancing overall performance of upconverted near-infrared circularly polarized luminescence for anticounterfeiting. ACS Nano 2023;17:2661-8.
35. Yang X, Jin X, Zhao T, Duan P. Circularly polarized luminescence in chiral nematic liquid crystals: generation and amplification. Mater Chem Front 2021;5:4821-32.
36. Eelkema R, Feringa BL. Amplification of chirality in liquid crystals. Org Biomol Chem 2006;4:3729-45.
37. Li X, Hu W, Wang Y, Quan Y, Cheng Y. Strong CPL of achiral AIE-active dyes induced by supramolecular self-assembly in chiral nematic liquid crystals (AIE-N*-LCs). Chem Commun 2019;55:5179-82.
38. Yoshida S, Morikawa S, Ueda K, Kaneko K, Hanasaki T, Akagi K. Helicity control of circularly polarized luminescence from aromatic conjugated copolymers and their mixture using reversibly photoinvertible chiral liquid crystals. ACS Appl Mater Interfaces 2024;16:3991-4002.
39. Lu X, Zhou Z, Ni B, et al. Tuning the circularly polarized luminescence of polymer-stabilized cholesteric liquid crystal films using chiral dopants. J Mater Chem C 2022;10:8246-53.
40. Gao J, Tian M, He Y, Yi H, Guo J. Multidimensional-encryption in emissive liquid crystal elastomers through synergistic usage of photorewritable fluorescent patterning and reconfigurable 3D shaping. Adv Funct Mater 2022;32:2107145.
41. Chen Y, Zhang Y, Li H, et al. Dynamic circularly polarized luminescence with tunable handedness and intensity enabled by achiral dichroic dyes in cholesteric liquid crystal medium. Adv Mater 2022;34:e2202309.
42. Wang X, Zhao B, Deng J. Liquid crystals doped with chiral fluorescent polymer: multi-color circularly polarized fluorescence and room-temperature phosphorescence with high dissymmetry factor and anti-counterfeiting application. Adv Mater 2023;35:e2304405.
43. Gong W, Zhou M, Xiao L, et al. Multicolor-tunable and time-dependent circularly polarized room-temperature phosphorescence from liquid crystal copolymers. Adv Opt Mater 2024;12:2301922.
44. Wu Y, Li M, Zheng ZG, Yu ZQ, Zhu WH. Liquid crystal assembly for ultra-dissymmetric circularly polarized luminescence and beyond. J Am Chem Soc 2023;145:12951-66.
46. Yuan J, Lu X, Lu Q. Large-scale chiral spherulites with controllable circularly polarized luminescence in liquid crystal block copolymers film. Aggregate 2024;5:e431.
47. Kang W, Tang Y, Meng X, et al. A photo- and thermo-driven azoarene-based circularly polarized luminescence molecular switch in a liquid crystal host. Angew Chem Int Ed Engl 2023;62:e202311486.
48. Wu Z, Pan K, Lü B, Ma L, Yang W, Yin M. Tunable morphology of spiropyran assemblies: from nanospheres to nanorods. Chem Asian J 2016;11:3102-6.
49. Shi Y, Han J, Jin X, Miao W, Zhang Y, Duan P. Chiral luminescent liquid crystal with multi-state-reversibility: breakthrough in advanced anti-counterfeiting materials. Adv Sci 2022;9:e2201565.
50. Kang S, Li Y, Bukharina D, et al. Bio-organic chiral nematic materials with adaptive light emission and on-demand handedness. Adv Mater 2021;33:e2103329.
51. Li RJ, Holstein JJ, Hiller WG, Andréasson J, Clever GH. Mechanistic interplay between light switching and guest binding in photochromic [Pd2Dithienylethene4] coordination cages. J Am Chem Soc 2019;141:2097-103.
52. Lin S, Gutierrez-cuevas KG, Zhang X, Guo J, Li Q. Fluorescent photochromic α-cyanodiarylethene molecular switches: an emerging and promising class of functional diarylethene. Adv Funct Mater 2021;31:2007957.
53. Lin S, Sun H, Qiao J, Ding X, Guo J. Phototuning energy transfer in self-organized luminescent helical superstructures for photonic applications. Adv Opt Mater 2020;8:2000107.
54. Li Y, Chen Y, Luo J, Quan Y, Cheng Y. Light-driven sign inversion of circularly polarized luminescence enabled by dichroism modulation in cholesteric liquid crystals. Adv Mater 2024;36:e2312331.
55. Sun J, Yu L, Wang L, et al. Optical intensity-driven reversible photonic bandgaps in self-organized helical superstructures with handedness inversion. J Mater Chem C 2017;5:3678-83.
56. Zhao D, van Leeuwen T, Cheng J, Feringa BL. Dynamic control of chirality and self-assembly of double-stranded helicates with light. Nat Chem 2017;9:250-6.
Cite This Article
How to Cite
Download Citation
Export Citation File:
Type of Import
Tips on Downloading Citation
Citation Manager File Format
Type of Import
Direct Import: When the Direct Import option is selected (the default state), a dialogue box will give you the option to Save or Open the downloaded citation data. Choosing Open will either launch your citation manager or give you a choice of applications with which to use the metadata. The Save option saves the file locally for later use.
Indirect Import: When the Indirect Import option is selected, the metadata is displayed and may be copied and pasted as needed.
About This Article
Copyright
Author Biographies
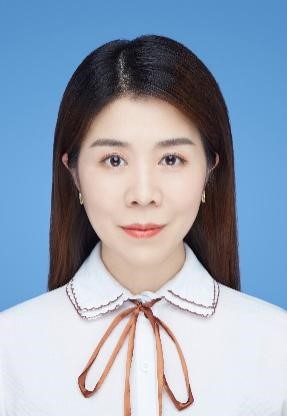
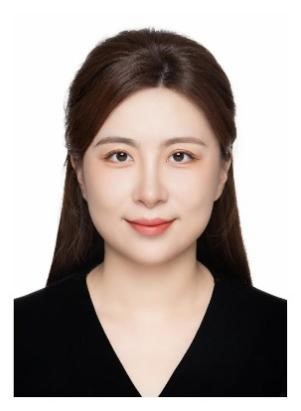
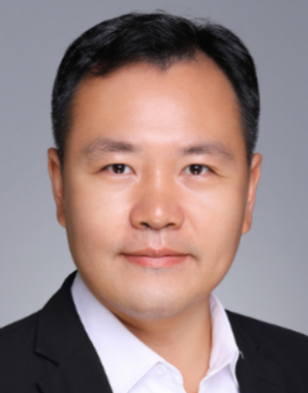
Data & Comments
Data
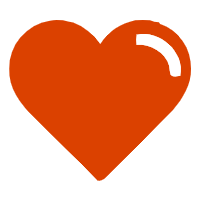
Comments
Comments must be written in English. Spam, offensive content, impersonation, and private information will not be permitted. If any comment is reported and identified as inappropriate content by OAE staff, the comment will be removed without notice. If you have any queries or need any help, please contact us at [email protected].