Organocatalytic Nazarov-type cyclization of 3-alkynyl-2-indolylmethanols: construction of axially chiral cyclopenta[b]indole scaffolds
Abstract
In recent years, it has become an urgent task to design new types of indole-based platform molecules for Nazarov-type cyclizations and develop organocatalytic Nazarov-type cyclizations for synthesizing indole derivatives. To fulfill this task, in this work, by changing the alkynyl terminal substituent from t-Bu to an aryl group, the reactivity of 3-alkynyl-2-indolylmethanols is modulated and the new platform molecules serve as competent substrates for Brønsted acid-catalyzed Nazarov-type cyclization. Based on this new reactivity, the first organocatalytic Nazarov-type cyclization of aryl-substituted 3-alkynyl-2-indolylmethanols with 2-naphthols is accomplished, leading to the efficient construction of a new class of axially chiral 3, 4-dihydrocyclopenta[b]indole scaffolds. This preliminary investigation of organocatalytic asymmetric Nazarov-type cyclization provides an optional strategy for the atroposelective construction of this new class of axially chiral cyclopenta[b]indole scaffolds. In addition, the first preparation of axially chiral 3, 4-dihydrocyclopenta[b]indole with optical purity is established through chiral resolution, which could serve as a complementary method to catalytic asymmetric approaches.
Keywords
INTRODUCTION
Indoles belong to an important nitrogen-containing heterocyclic motif that is present in many bioactive natural products and pharmaceuticals[1-4]. Therefore, the construction of indole-based frameworks, particularly via organocatalysis, has become an important field of study[5-8]. Among indole-fused rings, cyclopenta[b]indoles are attractive frameworks[9-13], which constitute the core structures of many natural products and biologically important compounds, such as yuehchukene[9], bruceolline I[10], fischerindole L[11], thomitrem A[12] and MK-0524[13] [Figure 1].
Figure 1. Representative natural products and bioactive compounds containing the cyclopenta[b]indole scaffold.
As a result, the construction of such indole-containing scaffolds has remained a long-standing goal in the chemistry community[14-20] and many synthetic approaches have been developed for the synthesis of these important structural units[21-29]. Among these approaches, the Nazarov-type cyclization[30-37] for the construction of 1, 2, 3, 4-tetrahydrocyclopenta[b]indole scaffolds is undoubtedly one of the most step-economical and efficient methods[38-47]. However, the classical synthesis of such indole derivatives via Nazarov-type cyclizations has largely focused on the Lewis acid (LA)-catalyzed 4π-electrocyclizations of indole-fused 1,4-dien-3-ones, which involve the process of generating a pentadienyl cation (I) intermediate to form the corresponding 1, 2, 3, 4-tetrahydrocyclopenta[b]indoles [Scheme 1A][37-41]. Nevertheless, other Nazarov-type cyclizations for the construction of such scaffolds are rather rare[42-47]. In our previous work, we designed 3-alkenyl-2-indolylmethanols as a new class of indole-based platform molecules for Brønsted acid-catalyzed interrupted Nazarov-type cyclizations with various nucleophiles[45-47] based on the formation of a pentadienyl cation (II) intermediate to construct 1, 2, 3, 4-tetrahydrocyclopenta[b]indole scaffolds [Scheme 1B]. In spite of these approaches, there are still some challenges in this research field. The first is that the indole-derived substrates suitable for Nazarov-type cyclizations are confined to indole-fused 1, 4-dien-3-ones and 3-alkenyl-2-indolylmethanols. The second is that many Nazarov-type cyclizations are enabled by Lewis acid catalysis and organocatalytic Nazarov-type cyclizations are underdeveloped[40,48-56], even though organocatalysis has been proven to have tremendous advantages[57-63]. Therefore, it has become an urgent task to design new types of indole-based platform molecules for Nazarov-type cyclizations and develop organocatalytic Nazarov-type cyclizations for synthesizing indole derivatives.
Scheme 1. Profile of the construction of 1, 2, 3, 4-tetrahydrocyclopenta[b]indole scaffolds via Nazarov-type cyclizations.
To overcome these challenges and fulfill this task, based on our long-lasting interests in synthesizing indole derivatives via designing indole-based platform molecules and their involved organocatalytic reactions[5-8], we decided to design a new type of indole-based platform molecules for organocatalytic Nazarov-type cyclizations. In our previous work, we designed t-Bu-substituted 3-alkynyl-2-indolylmethanols for constructing axially chiral alkene-indole scaffolds via addition reactions [Scheme 2A]. Specifically, in the presence of a chiral Brønsted acid, this class of 3-alkynyl-2-indolylmethanols transformed into allene-iminium intermediates, which were readily attacked by nucleophiles to undergo 1, 4-addition, thus giving axially chiral alkene-indoles. When using dinucleophiles, the OH group of 2-indolylmethanols undergoes dehydration to give carbocation intermediates[64-72], which subsequently undergo an intramolecular addition reaction to generate axially chiral cyclic alkene-indoles[73,74]. In these previous studies, the t-Bu group, as an aliphatic and bulky group, was detrimental to the delocalization of carbocation, thus making this class of 3-alkynyl-2-indolylmethanols unsuitable for Nazarov-type cyclizations. On this basis, we considered changing the t-Bu group to a less steric aryl (Ar) group, thus making aryl-substituted 3-alkynyl-2-indolylmethanols suitable for Nazarov-type cyclizations [Scheme 2B]. This design is based on the consideration that the carbocation can readily undergo 4π electron delocalization due to the existence of the terminal aryl group, therefore undergoing Nazarov-type cyclization and constructing 3, 4-dihydro-cyclopenta[b]indoles.
Scheme 2. Design of a new type of 3-alkynyl-2-indolylmethanols for constructing 3, 4-dihydrocyclopenta
Based on this concept, we design an organocatalytic Nazarov-type cyclization of aryl-substituted 3-alkynyl-2-indolylmethanols with 2-naphthols [Scheme 2C]. The selection of 2-naphthols as suitable nucleophiles is based on the consideration that 2-naphthols[75-77] are easily activated by Brønsted acids through the interaction of hydrogen bonding. It is noteworthy that 2-naphthols with a planar structure and the effect of steric congestion should lead to the formation of a C-C bond as a chiral axis[78-80], thus endowing the constructed cyclopenta[b]indole frameworks with axial chirality[81-89]. Therefore, the significance of this work is threefold: (1) modulating the terminal substituents of 3-alkynyl-2-indolylmethanols to achieve different reactivities; (2) the first organocatalytic Nazarov-type cyclization of aryl-substituted 3-alkynyl-2-indolylmethanols; (3) the efficient construction of a new class of axially chiral 3, 4-dihydrocyclopenta
EXPERIMENTAL
To a mixture of 3-phenyl-2-indolylmethanol 1 (0.30 mmol), 2-naphthol 2 (0.2 mmol) and catalyst 4a (7.0 mg, 0.02 mmol) was added CHCl3 (1 mL). The reaction mixture was then stirred at 30 °C for 12 h. After the completion of the reaction, which was indicated by thin layer chromatography, the reaction mixture was directly purified through column chromatography on silica gel (petroleum ether:dichloromethane = 2:1 as eluent) to afford pure product 3.
RESULTS AND DISCUSSION
Based on this design, we initially attempted the reaction of 3-alkynyl-2-indolylmethanol 1a bearing a terminal phenyl group with 2-naphthol 2a in the presence of 10 mol% racemic phosphoric acid 4a in chloroform (CHCl3) at 20 °C for 12 h [Table 1 and entry 1]. Gratifyingly, the designed Nazarov-type cyclization occurred in a facile manner to afford cyclopenta[b]indole 3aa in a moderate yield of 55%. A series of Brønsted acids 4 were then evaluated for the reaction (entries 2-7), which revealed that p-toluenesulfonic acid monohydrate 4c (TsOH.H2O) and trifluoromethanesulfonic acid 4d (TfOH) could catalyze the reaction to some extent (entries 3 and 4), whereas other Brønsted acids could barely catalyze the reaction and only trace amounts of product were observed (entries 2 and 5-7). Therefore, racemic phosphoric acid 4a was selected as the optimal catalyst for this Nazarov-type cyclization. Subsequently, several different types of solvents were screened (entries 8-12). It was found that the reaction could only occur in chloroform (entry 1) and toluene (entry 8), with chloroform acting as a better reaction medium in terms of yield. To further improve the yield of this model reaction, other reaction parameters, such as reagent ratio and reaction concentration and temperature, were modulated (entries 13-20). It was found that the yield of product 3aa could be improved by modulating the ratio of 1a and 2a (entries 13-15). When the ratio was adjusted to 1.5:1, the yield of product 3aa could be increased to 70% (entry 15). In addition, the subsequent evaluation of the reaction concentration (entries 16-18) revealed that a higher concentration was helpful for increasing the yield (entry 16), i.e., when the reaction was performed in 0.5 mL CHCl3, product 3aa could be obtained in a higher yield of 76% (entry 16). Finally, slightly modulating the reaction temperature (entries 19 and 20) resulted in the yield of product 3aa being further improved to 85% when performing the reaction at 30 °C (entry 19). Therefore, the optimal conditions for the Nazarov-type cyclization were set as those of entry 19.
Screening of catalysts and optimization of reaction conditionsa
![]() | |||||
Entry | Cat. | Solvent | T (oC) | 1a: 2a | Yield (%)b |
1 | 4a | CHCl3 | 20 | 1:1.2 | 55 |
2 | 4b | CHCl3 | 20 | 1:1.2 | trace |
3 | 4c | CHCl3 | 20 | 1:1.2 | 45 |
4 | 4d | CHCl3 | 20 | 1:1.2 | 11 |
5 | 4e | CHCl3 | 20 | 1:1.2 | trace |
6 | 4f | CHCl3 | 20 | 1:1.2 | trace |
7 | 4g | CHCl3 | 20 | 1:1.2 | trace |
8 | 4a | toluene | 20 | 1:1.2 | 43 |
9 | 4a | acetone | 20 | 1:1.2 | trace |
10 | 4a | MeCN | 20 | 1:1.2 | trace |
11 | 4a | THF | 20 | 1:1.2 | trace |
12 | 4a | EtOAc | 20 | 1:1.2 | trace |
13 | 4a | CHCl3 | 20 | 1:1.5 | 64 |
14 | 4a | CHCl3 | 20 | 1.2:1 | 62 |
15 | 4a | CHCl3 | 20 | 1.5:1 | 70 |
16c | 4a | CHCl3 | 20 | 1.5:1 | 76 |
17d | 4a | CHCl3 | 20 | 1.5:1 | 61 |
18e | 4a | CHCl3 | 20 | 1.5:1 | 37 |
19c | 4a | CHCl3 | 30 | 1.5:1 | 85 |
20c | 4a | CHCl3 | 40 | 1.5:1 | 79 |
With the optimal reaction conditions determined, we then investigated the substrate scope of the Nazarov-type cyclization [Figure 2]. First, the substrate scope of the 3-alkynyl-2-indolylmethanols 1 was studied by reactions with indole 2a. As shown in Figure 2, the Brønsted acid-catalyzed Nazarov-type cyclization was compatible with a variety of substrates 1 bearing different R/Ar/Ar1 substituents, which successfully participated in the reaction to give the expected 3, 4-dihydrocyclopenta
Figure 2. Substrate scope of Nazarov-type cyclization. Reaction conditions: 0.2 mmol scale; 10 mol% 4a; CHCl3 (1.0 mL); 30 ℃; 12 h; 1:2 = 1.5:1. Isolated yields.
Next, the substrate scope of 2-naphthols 2 was investigated by the Nazarov-type cyclization with 3-alkynyl-2-indolylmethanol 1a. As shown in Figure 2, this reaction was amenable to a series of C6- and C7-substituted 2-naphthols 2b-2i, which underwent the Nazarov-type cyclization to afford the desired products 3ab-3ai in moderate to good yields. In detail, C6-substituted 2-naphthols 2, regardless of their electronic nature, could be applicable to the reaction, and it was found that 2-naphthol 2c with an electron-donating group could give product 3ac in the highest yield of 86%. For C7-substituted 2-naphthols 2, various substituents with electron-donating or electron-withdrawing properties were tolerant to the reaction and 2-naphthol 2g with a C7-methoxyl group could furnish product 3ag in a high yield of 81%. Interestingly, 2-naphthalenethiol 2j serving as an analogue of 2-naphthol 2a could be employed for the Nazarov-type cyclization under the standard conditions, giving product 3aj in a moderate yield.
In addition, we performed a 1 mmol scale reaction of 3-alkynyl-2-indolylmethanol 1a with 2-naphthol 2a under the optimal reaction conditions [Scheme 3A]. In this case, product 3aa was afforded in a high yield of 80%, which demonstrated that this Brønsted acid-catalyzed Nazarov-type cyclization could be scaled up and should have potential applications. In order to gain some insights into the organocatalytic Nazarov-type cyclization, we performed some control experiments [Scheme 3B]. First, N-methyl-protected 3-alkynyl-2-indolylmethanol 1o was employed as a substrate to the reaction with 2-naphthol 2a under the standard reaction conditions with no reaction observed, which indicated that the NH group of 3-alkynyl-2-indolylmethanol 1 played an important role in controlling the reactivity. Second, O-methyl-protected substrate 2k was used as a nucleophile to react with 1a and still no reaction occurred. This result demonstrated that the OH group of substrate 2 was necessary for performing the reaction.
Based on the control experiments, a possible reaction pathway and activation mode of this Brønsted acid-catalyzed reaction were proposed [Scheme 4]. As exemplified by the model reaction, 3-alkynyl-2-indolylmethanol 1a was initially transformed into allene-iminium intermediate A under the activation of Brønsted acid 4a via hydrogen-bonding interaction. Subsequently, catalyst 4a simultaneously activated allene-iminium intermediate A and 2-naphthol 2a via forming two hydrogen bonds, thus facilitating a 1, 4-addition between them to generate intermediate B. Intermediate B then experienced a dehydration process under the catalysis of Brønsted acid 4a to give carbocation C, which was easily converted into 4π carbocation D due to electron delocalization. Finally, activated by catalyst 4a via the interactions of hydrogen bonding and ion pairing, intermediate D underwent a Nazarov-type cyclization to form the cyclic carbocation intermediate E, which immediately underwent α-H elimination to deliver 3, 4-dihydrocyclopenta
Because this class of 3, 4-dihydrocyclopenta
Preliminary investigation of organocatalytic asymmetric version of Nazarov-type cyclizationa
![]() | ||||
Entry | Cat. | Solvent | Yield (%)b | ee (%)c |
1 | 5a | CHCl3 | 85 | 12 |
2 | 5b | CHCl3 | 32 | 10 |
3 | 5c | CHCl3 | trace | - |
4 | 5d | CHCl3 | 21 | 27 |
5 | 5e | CHCl3 | 10 | 4 |
6 | 5f | CHCl3 | 7 | 47 |
7 | 5g | CHCl3 | trace | - |
8 | 5h | CHCl3 | 89 | 2 |
9 | 5i | CHCl3 | 47 | 10 |
10 | 6a | CHCl3 | 58 | 15 |
11 | 6b | CHCl3 | trace | - |
12 | 7a | CHCl3 | 34 | 16 |
13 | 7b | CHCl3 | trace | - |
14 | 5f | toluene | 11 | 69 |
15 | 5f | EtOAc | trace | - |
16 | 5f | THF | trace | - |
17 | 5f | MeCN | trace | - |
18 | 5f | acetone | trace | - |
19d | 5f | toluene | 9 | 68 |
20e | 5f | toluene | 20 | 69 |
Finally, to obtain the two enantiomers of axially chiral 3aa, we tried using the strategy of chiral resolution [Scheme 5]. In detail, the racemic compound rac-3aa was subjected to the acylation reaction with (R)-(-)-O-formylmandeloyl chloride (R)-8 as a resolution reagent in the presence of DMAP, which gave rise to two separable diastereomers (Sa, R)-9 and (Ra, R)-9. By treating with hydrazine hydrate, the two diastereomers were then easily transformed into the corresponding single enantiomers (Sa)-3aa and (Ra)-3aa, respectively, in high yields with excellent atroposelectivities. In this manner, the first preparation of axially chiral 3, 4-dihydrocyclopenta
CONCLUSION
In summary, by changing the alkynyl terminal substituent from t-Bu to an aryl group, the reactivity of 3-alkynyl-2-indolylmethanols was modulated as competent platform molecules for Brønsted acid-catalyzed Nazarov-type cyclization. Based on this new reactivity, we accomplished the first organocatalytic Nazarov-type cyclization of aryl-substituted 3-alkynyl-2-indolylmethanols with 2-naphthols, thus realizing the efficient construction of a new class of axially chiral 3, 4-dihydrocyclopenta
DECLARATIONS
AcknowledgmentsWe sincerely thank all the group members who are involved in the development of new kinds of indolylmethanols as platform molecules.
Authors’ contributionsPerforming the majority of the experiments: Wu P
Doing some of the experiments: Yan XY, Jiang S
Initially trying the model reaction: Lu YN
Co-directing this project and writing the draft manuscript: Tan W
Directing this project and revising the manuscript: Shi F
Availability of data and materialsSupplementary Materials are available online for this paper.
Financial support and sponsorshipWe thank National Natural Science Foundation of China (22125104 and 21831007), Natural Science Foundation of Jiangsu Province (BK20210916) and High Education Natural Science Foundation of Jiangsu Province (No. 21KJB150009) for financial support.
Conflicts of interestThe author declared that there is no conflict of interest.
Ethical approval and consent to participateNot applicable.
Consent for publicationNot applicable.
Copyright© The Author(s) 2023.
Supplementary MaterialsREFERENCES
1. Sundberg RJ. The chemistry of indoles. New York: Academic Press; 1996.
2. Ryan KS, Drennan CL. Divergent pathways in the biosynthesis of bisindole natural products. Chem Biol 2009;16:351-64.
3. Kochanowska-Karamyan AJ, Hamann MT. Marine indole alkaloids: potential new drug leads for the control of depression and anxiety. Chem Rev 2010;110:4489-97.
4. Ishikura M, Abe T, Choshi T, Hibino S. Simple indole alkaloids and those with a non-rearranged monoterpenoid unit. Nat Prod Rep 2013;30:694-752.
5. Zhang YC, Jiang F, Shi F. Organocatalytic asymmetric synthesis of indole-based chiral heterocycles: strategies, reactions, and outreach. Acc Chem Res 2020;53:425-46.
6. Sheng F, Wang J, Tan W, Zhang Y, Shi F. Progresses in organocatalytic asymmetric dearomatization reactions of indole derivatives. Org Chem Front 2020;7:3967-98.
7. Tu M, Chen K, Wu P, Zhang Y, Liu X, Shi F. Advances in organocatalytic asymmetric reactions of vinylindoles: powerful access to enantioenriched indole derivatives. Org Chem Front 2021;8:2643-72.
8. Zhang HH, Shi F. Organocatalytic atroposelective synthesis of indole derivatives bearing axial chirality: strategies and applications. Acc Chem Res 2022;55:2562-80.
11. Park A, Moore RE, Patterson GM. Fischerindole L, a new isonitrile from the terrestrial blue-green alga fischerella muscicola. Tetrahedron Lett 1992;33:3257-60.
12. Rundberget T, Wilkins AL. Thomitrems A and E, two indole-alkaloid isoprenoids from penicillium crustosum thom. Phytochemistry 2002;61:979-85.
13. Nicoll-Griffith DA, Seto C, Aubin Y, et al. In vitro biotransformations of the prostaglandin D2 (DP) antagonist MK-0524 and synthesis of metabolites. Bioorg Med Chem Lett 2007;17:301-4.
14. Tan W, Li X, Gong YX, Ge MD, Shi F. Highly diastereo- and enantioselective construction of a spiro[cyclopenta[
15. Shi F, Zhang HH, Sun XX, Liang J, Fan T, Tu SJ. Organocatalytic asymmetric cascade reactions of 7-vinylindoles: diastereo- and enantioselective synthesis of C7-functionalized indoles. Chem Eur J 2015;21:3465-71.
16. Gandhi S, Baire B. Unusual formation of cyclopenta[
17. Selvaraj K, Debnath S, Swamy KCK. Reaction of indole carboxylic acid/amide with propargyl alcohols: [4+3]-annulation, unexpected 3- to 2- carboxylate/amide migration, and decarboxylative cyclization. Org Lett 2019;21:5447-51.
18. Yadav S, Hazra R, Singh A, Ramasastry SSV. Substituent-guided palladium-ene reaction for the synthesis of carbazoles and cyclopenta[
19. Agy AC, Rodrigues Jr. MT, Zeoly LA, Simoni DA, Coelho F. Palladium-mediated oxidative annulation of δ-indolyl-α,β-unsaturated compounds toward the synthesis of cyclopenta[
20. Yu KY, Ge XM, Fan YJ, et al. Iron(III)-catalyzed tandem annulation of indolyl-substituted
21. Duan S, Zhang W, Hu Y, Xu ZF, Li CY. Synthesis of cyclopenta[
22. Jadhav SD, Singh T, Singh A. Brønsted acid promoted C-C bond formation between indolylmethyl electrophiles and ketene dithioacetals: diastereoselective synthesis of highly functionalized cyclopenta[
23. Mao J, Zhang H, Ding XF, Luo X, Deng WP. Synergistic catalysis for asymmetric [3+2] cycloadditions of 2-indolylmethanols with α, β-unsaturated aldehydes. J Org Chem 2019;84:11186-94.
24. Tian F, Yang W, Ni T, Zhang J, Deng W. Catalytic asymmetric dipolar cycloadditions of indolyl delocalized metal-allyl species for the enantioselective synthesis of cyclopenta[
25. Gao JG, Guan XK, Sun DY, et al. Enantioselective domino reaction of 3-vinylindole and
26. Fan T, Zhang H, Li C, Shen Y, Shi F. The application of
27. Zhang H, Zhu Z, Fan T, Liang J, Shi F. Intermediate-dependent unusual [4+3], [3+2] and cascade reactions of 3-indolylmethanols: controllable chemodivergent and stereoselective synthesis of diverse indole derivatives. Adv Synth Catal 2016;358:1259-88.
28. Zhu Z, Shen Y, Sun X, Tao J, Liu J, Shi F. Catalytic asymmetric [3+2] cycloadditions of C-3 unsubstituted 2-indolylmethanols: regio-, diastereo- and enantioselective construction of the cyclopenta[
29. Xu MM, Wang HQ, Wan Y, Wang SL, Shi F. Enantioselective construction of cyclopenta[
30. Vaidya T, Eisenberg R, Frontier AJ. Catalytic Nazarov cyclization: the state of the art. ChemCatChem 2011;3:1531-48.
34. Wenz DR, Read de Alaniz J. The Nazarov cyclization: a valuable method to synthesize fully substituted carbon stereocenters: Nazarov cyclization. Eur J Org Chem 2015;2015:23-37.
35. Simeonov SP, Nunes JP, Guerra K, Kurteva VB, Afonso CA. Synthesis of chiral cyclopentenones. Chem Rev 2016;116:5744-893.
36. Vinogradov MG, Turova OV, Zlotin SG. Nazarov reaction: current trends and recent advances in the synthesis of natural compounds and their analogs. Org Biomol Chem 2017;15:8245-69.
37. Malona JA, Colbourne JM, Frontier AJ. A general method for the catalytic Nazarov cyclization of heteroaromatic compounds. Org Lett 2006;8:5661-4.
38. Davies J, Leonori D. The first calcium-catalysed Nazarov cyclisation. Chem Commun 2014;50:15171-4.
39. Raja S, Nakajima M, Rueping M. Experimental and computational study of the catalytic asymmetric 4π-electrocyclization of
40. Wang GP, Chen MQ, Zhu SF, Zhou QL. Enantioselective Nazarov cyclization of indole enones cooperatively catalyzed by Lewis acids and chiral Brønsted acids. Chem Sci 2017;8:7197-202.
41. Rinaldi A, Petrović M, Magnolfi S, Scarpi D, Occhiato EG. Pentannulation reaction by tandem gold(I)-catalyzed propargyl Claisen rearrangement/Nazarov cyclization of enynyl vinyl ethers. Org Lett 2018;20:4713-7.
42. Dhiman S, Ramasastry SS. One-pot relay gold(I) and brønsted acid catalysis: cyclopenta[
43. Wang Z, Xu X, Gu Z, et al. Nazarov cyclization of 1,4-pentadien-3-ols: preparation of cyclopenta[
44. Scarpi D, Petrović M, Fiser B, Gómez-Bengoa E, Occhiato EG. Construction of Cyclopenta[
45. Wang C, Wu J, Li C, Li L, Mei G, Shi F. Design of C3-alkenyl-substituted 2-indolylmethanols for catalytic asymmetric interrupted Nazarov-type cyclization. Adv Synth Catal 2018;360:846-51.
46. Wu JL, Wang JY, Wu P, Wang JR, Mei GJ, Shi F. Diastereo- and enantioselective construction of chiral cyclopenta[
47. Wang JY, Wu P, Wu JL, Mei GJ, Shi F. Chemodivergent tandem cyclizations of 2-indolylmethanols with tryptophols: C-N versus C-C bond formation. J Org Chem 2018;83:5931-46.
48. Rueping M, Ieawsuwan W, Antonchick AP, Nachtsheim BJ. Chiral Brønsted acids in the catalytic asymmetric Nazarov cyclization--the first enantioselective organocatalytic electrocyclic reaction. Angew Chem Int Ed Engl 2007;46:2097-100.
49. Rueping M, Ieawsuwan W. A catalytic asymmetric electrocyclization-protonation reaction. Adv Synth Catal 2009;351:78-84.
50. Basak AK, Shimada N, Bow WF, Vicic DA, Tius MA. An organocatalytic asymmetric Nazarov cyclization. J Am Chem Soc 2010;132:8266-7.
51. Jolit A, Walleser PM, Yap GP, Tius MA. Catalytic enantioselective Nazarov cyclization: construction of vicinal all-carbon-atom quaternary stereocenters. Angew Chem Int Ed Engl 2014;53:6180-3.
52. Yang BM, Cai PJ, Tu YQ, et al. Organocatalytic asymmetric tandem Nazarov cyclization/semipinacol rearrangement: rapid construction of chiral spiro[4.4]nonane-1,6-diones. J Am Chem Soc 2015;137:8344-7.
53. Süsse L, Vogler M, Mewald M, Kemper B, Irran E, Oestreich M. Enantioselective Nazarov cyclizations catalyzed by an axial chiral C6F5 -substituted boron Lewis acid. Angew Chem Int Ed Engl 2018;57:11441-4.
54. Ouyang J, Kennemur JL, De CK, Farès C, List B. Strong and confined acids enable a catalytic asymmetric Nazarov cyclization of simple divinyl ketones. J Am Chem Soc 2019;141:3414-8.
55. Cao J, Hu MY, Liu SY, Zhang XY, Zhu SF, Zhou QL. Enantioselective silicon-directed Nazarov cyclization. J Am Chem Soc 2021;143:6962-8.
56. Cao J, Zhu S. Chiral proton-transfer shuttle catalysts promoted enantioselective Nazarov cyclization. Synlett 2023;34:29-39.
59. Wang T, Han X, Zhong F, Yao W, Lu Y. Amino acid-derived bifunctional phosphines for enantioselective transformations. Acc Chem Res 2016;49:1369-78.
60. Zong L, Tan CH. Phase-transfer and ion-pairing catalysis of pentanidiums and bisguanidiniums. Acc Chem Res 2017;50:842-56.
61. Ni H, Chan WL, Lu Y. Phosphine-catalyzed asymmetric organic reactions. Chem Rev 2018;118:9344-411.
62. Metrano AJ, Miller SJ. Peptide-based catalysts reach the outer sphere through remote desymmetrization and atroposelectivity. Acc Chem Res 2019;52:199-215.
63. Tan W, Zhang JY, Gao CH, Shi F. Progress in organocatalytic asymmetric (4+3) cycloadditions for the enantioselective construction of seven-membered rings. Sci China Chem 2023;Epub ahead of print.
64. Mei GJ, Shi F. Indolylmethanols as reactants in catalytic asymmetric reactions. J Org Chem 2017;82:7695-707.
65. Petrini M. New perspectives in the indole ring functionalization using 2-indolylmethanols. Adv Synth Catal 2020;362:1214-32.
66. Zhang H, Shi F. Advances in catalytic asymmetric reactions using 2-indolylmethanols as platform molecules. Chinese J Org Chem 2022;42:3351.
67. Tan W, Shi F. A breakthrough in 2-indolylmethanol-involved organocatalytic asymmetric reactions. Chem Synth 2022;2:11.
68. Bera K, Schneider C. Brønsted acid catalyzed [3+2]-cycloaddition of 2-vinylindoles with in situ generated 2-methide-2
69. Göricke F, Schneider C. Palladium-catalyzed, enantioselective (3+2)-cycloannulation of
70. Li X, Duan M, Deng Z, et al. Catalytic enantioselective synthesis of chiral tetraarylmethanes. Nat Catal 2020;3:1010-9.
71. Li X, Sun J. Organocatalytic enantioselective synthesis of chiral allenes: remote asymmetric 1, 8-addition of indole imine methides. Angew Chem Int Ed Engl 2020;59:17049-54.
72. Li X, Duan M, Yu P, Houk KN, Sun J. Organocatalytic enantioselective dearomatization of thiophenes by 1, 10-conjugate addition of indole imine methides. Nat Commun 2021;12:4881.
73. Wang J, Sun M, Yu X, Zhang Y, Tan W, Shi F. Atroposelective construction of axially chiral alkene-indole scaffolds via catalytic enantioselective addition reaction of 3-alkynyl -2-indolylmethanols. Chin J Chem 2021;39:2163-71.
74. Wang C, Li T, Liu S, et al. Axially chiral aryl-alkene-indole framework: a nascent member of the atropisomeric family and its catalytic asymmetric construction. Chin J Chem 2020;38:543-52.
75. Zhang HH, Wang CS, Li C, Mei GJ, Li Y, Shi F. Design and enantioselective construction of axially chiral naphthyl-indole skeletons. Angew Chem Int Ed Engl 2017;56:116-21.
76. Wang Y, Yu P, Zhou Z, et al. Rational design, enantioselective synthesis and catalytic applications of axially chiral EBINOLs. Nat Catal 2019;2:504-13.
77. Ding W, Yu P, An Q, et al. DFT-guided phosphoric-acid-catalyzed atroposelective arene functionalization of nitrosonaphthalene. Chem 2020;6:2046-59.
78. Li TZ, Liu SJ, Tan W, Shi F. Catalytic asymmetric construction of axially chiral indole-based frameworks: an emerging area. Chem Eur J 2020;26:15779-92.
79. Cheng JK, Xiang SH, Li S, Ye L, Tan B. Recent advances in catalytic asymmetric construction of atropisomers. Chem Rev 2021;121:4805-902.
80. Bai XF, Cui YM, Cao J, Xu LW. Atropisomers with axial and point chirality: synthesis and applications. Acc Chem Res 2022;55:2545-61.
81. Wang J, Zhao C, Wang J. Recent progress toward the construction of axially chiral molecules catalyzed by an
82. Song R, Xie Y, Jin Z, Chi YR. Carbene-catalyzed asymmetric construction of atropisomers. Angew Chem Int Ed Engl 2021;60:26026-37.
83. Zhang Z, Zhai T, Ye L. Synthesis of axially chiral compounds through catalytic asymmetric reactions of alkynes. Chem Catalysis 2021;1:1378-412.
84. Liu CX, Zhang WW, Yin SY, Gu Q, You SL. Synthesis of atropisomers by transition-metal-catalyzed asymmetric C-H functionalization reactions. J Am Chem Soc 2021;143:14025-40.
85. Zhang X, Zhao K, Gu Z. Transition metal-catalyzed biaryl atropisomer synthesis via a torsional strain promoted ring-opening reaction. Acc Chem Res 2022;55:1620-33.
86. Qin W, Liu Y, Yan H. Enantioselective synthesis of atropisomers via vinylidene
87. Basilaia M, Chen MH, Secka J, Gustafson JL. Atropisomerism in the pharmaceutically relevant realm. Acc Chem Res 2022;55:2904-19.
88. Cheng JK, Xiang SH, Tan B. Organocatalytic enantioselective synthesis of axially chiral molecules: development of strategies and skeletons. Acc Chem Res 2022;55:2920-37.
89. Kumar A, Sasai H, Takizawa S. Atroposelective synthesis of C-C axially chiral compounds via mono- and dinuclear vanadium catalysis. Acc Chem Res 2022;55:2949-65.
90. Norton RS, Wells RJ. A series of chiral polybrominated biindoles from the marine blue-green alga Rivularia firma. Application of carbon-13 NMR spin-lattice relaxation data and carbon-13-proton coupling constants to structure elucidation. J Am Chem Soc 1982;104:3628-35.
91. Ito C, Thoyama Y, Omura M, Kajiura I, Furukawa H. Alkaloidal constituents of murraya koenigii. isolation and structural elucidation of novel binary carbazolequinones and carbazole alkaloids. Chem Pharm Bull 1993;41:2096-100.
92. Zhang Q, Mándi A, Li S, et al. N-N-coupled indolo-sesquiterpene atropo-diastereomers from a marine-derived actinomycete. Eur J Org Chem 2012;2012:5256-62.
93. Jiang F, Chen KW, Wu P, Zhang YC, Jiao Y, Shi F. A strategy for synthesizing axially chiral naphthyl-indoles: catalytic asymmetric addition reactions of racemic substrates. Angew Chem Int Ed Engl 2019;58:15104-10.
94. Chen KW, Chen ZH, Yang S, Wu SF, Zhang YC, Shi F. Organocatalytic atroposelective synthesis of
95. Xia W, An QJ, Xiang SH, Li S, Wang YB, Tan B. Chiral phosphoric acid catalyzed atroposelective C-H amination of arenes. Angew Chem Int Ed Engl 2020;59:6775-9.
96. Baumann T, Brückner R. Atropselective dibrominations of a 1, 1'-disubstituted 2, 2'-biindolyl with diverging point-to-axial asymmetric inductions. Deriving 2, 2'-biindolyl-3, 3'-diphosphane ligands for asymmetric catalysis. Angew Chem Int Ed Engl 2019;58:4714-9.
97. He T, Peng L, Li S, et al. Chiral naphthyl-C2-indole as scaffold for phosphine organocatalysis: application in asymmetric formal [4+2] cycloaddition reactions. Org Lett 2020;22:6966-71.
98. Wang L, Zhong J, Lin X. Atroposelective phosphoric acid catalyzed three-component cascade reaction: enantioselective synthesis of axially chiral
99. Ren Q, Cao T, He C, Yang M, Liu H, Wang L. Highly atroposelective rhodium(II)-catalyzed N-H bond insertion: access to axially chiral
100. Wang ZS, Zhu LJ, Li CT, Liu BY, Hong X, Ye LW. Synthesis of axially chiral
101. Yang H, Sun HR, He RQ, et al. Organocatalytic cycloaddition of alkynylindoles with azonaphthalenes for atroposelective construction of indole-based biaryls. Nat Commun 2022;13:632.
102. Ototake N, Morimoto Y, Mokuya A, Fukaya H, Shida Y, Kitagawa O. Catalytic enantioselective synthesis of atropisomeric indoles with an N-C chiral axis. Chem Eur J 2010;16:6752-5.
103. Zhang J, Xu Q, Wu J, Fan J, Xie M. Construction of N-C axial chirality through atroposelective C-H olefination of
104. Sun L, Chen H, Liu B, et al. Rhodium-catalyzed atroposelective construction of indoles via C-H bond activation. Angew Chem Int Ed Engl 2021;60:8391-5.
105. Kim A, Kim A, Park S, et al. Catalytic and enantioselective control of the C-N stereogenic axis via the Pictet-Spengler reaction. Angew Chem Int Ed Engl 2021;60:12279-83.
106. Corti V, Thøgersen MK, Enemaerke VJ, Rezayee NM, Barløse CL, Anker Jørgensen K. Construction of C-N atropisomers by aminocatalytic enantioselective addition of indole-2-carboxaldehydes to
107. Li Y, Liou YC, Chen X, Ackermann L. Thioether-enabled palladium-catalyzed atroposelective C-H olefination for N-C and C-C axial chirality. Chem Sci 2022;13:4088-94.
108. Wang Y, Zhou X, Shan W, et al. Construction of axially chiral indoles by cycloaddition-isomerization via atroposelective phosphoric acid and silver sequential catalysis. ACS Catal 2022;12:8094-103.
109. Wang P, Huang Y, Jing J, Wang F, Li X. Rhodium(III)-catalyzed atroposelective synthesis of C-N axially chiral naphthylamines and variants via C-H activation. Org Lett 2022;24:2531-5.
110. Niu C, Zhou Y, Chen Q, et al. Atroposelective synthesis of
111. Qi LW, Mao JH, Zhang J, Tan B. Organocatalytic asymmetric arylation of indoles enabled by azo groups. Nat Chem 2018;10:58-64.
112. Bisag GD, Pecorari D, Mazzanti A, et al. Central-to-axial chirality conversion approach designed on organocatalytic enantioselective povarov cycloadditions: first access to configurationally stable indole-quinoline atropisomers. Chem Eur J 2019;25:15694-701.
113. Mao JH, Wang YB, Yang L, et al. Organocatalyst-controlled site-selective arene C-H functionalization. Nat Chem 2021;13:982-91.
114. Xu WL, Zhao WM, Zhang RX, Chen J, Zhou L. Organocatalytic cycloaddition-elimination cascade for atroposelective construction of heterobiaryls. Chem Sci 2021;12:14920-6.
115. He C, Hou M, Zhu Z, Gu Z. Enantioselective synthesis of indole-based biaryl atropisomers via palladium-catalyzed dynamic kinetic intramolecular C-H cyclization. ACS Catal 2017;7:5316-20.
116. Xi CC, Zhao XJ, Tian JM, et al. Atroposelective synthesis of axially chiral 3-arylindoles by copper-catalyzed asymmetric cross-coupling of indoles with quinones and naphthoquinones. Org Lett 2020;22:4995-5000.
117. Shaaban S, Li H, Otte F, Strohmann C, Antonchick AP, Waldmann H. Enantioselective synthesis of five-membered-ring atropisomers with a chiral Rh(III) complex. Org Lett 2020;22:9199-202.
118. Li X, Zhao L, Qi Z, Li X. Construction of atropisomeric 3-arylindoles via enantioselective Cacchi reaction. Org Lett 2021;23:5901-5.
119. Wang CS, Wei L, Fu C, Wang XH, Wang CJ. Asymmetric synthesis of axially chiral naphthyl-C3-indoles via a palladium-catalyzed Cacchi reaction. Org Lett 2021;23:7401-6.
120. Surgenor RR, Liu X, Keenlyside MJH, Myers W, Smith MD. Enantioselective synthesis of atropisomeric indoles via iron-catalysed oxidative cross-coupling. Nat Chem 2022; doi: 10.1038/s41557-022-01095-9.
121. Hu YL, Wang Z, Yang H, et al. Conversion of two stereocenters to one or two chiral axes: atroposelective synthesis of 2, 3-diarylbenzoindoles. Chem Sci 2019;10:6777-84.
122. Peng L, Li K, Xie C, et al. Organocatalytic asymmetric annulation of
123. He YP, Wu H, Wang Q, Zhu J. Palladium-catalyzed enantioselective Cacchi reaction: asymmetric synthesis of axially chiral 2, 3-disubstituted indoles. Angew Chem Int Ed Engl 2020;59:2105-9.
124. Xu D, Huang S, Hu F, et al. Diversity-oriented enantioselective construction of atropisomeric heterobiaryls and
125. Jia S, Tian Y, Li X, Wang P, Lan Y, Yan H. Atroposelective construction of nine-membered carbonate-bridged biaryls. Angew Chem Int Ed Engl 2022;61:e202206501.
126. Yin SY, Pan C, Zhang WW, et al. SCpRh(III)-catalyzed enantioselective synthesis of atropisomers by C2-arylation of indoles with 1-diazonaphthoquinones. Org Lett 2022;24:3620-5.
127. Liu J, Li Q, Shao Y, Sun J. Atroposelective synthesis of axially chiral C2-arylindoles via rhodium-catalyzed asymmetric C-H bond insertion. Org Lett 2022;24:4670-4.
128. Zhu S, Chen YH, Wang YB, et al. Organocatalytic atroposelective construction of axially chiral arylquinones. Nat Commun 2019;10:4268.
129. Chen YH, Li HH, Zhang X, Xiang SH, Li S, Tan B. Organocatalytic enantioselective synthesis of atropisomeric aryl-
130. Hang Q, Wu S, Yang S, et al. Design and catalytic atroposelective synthesis of axially chiral isochromenone-indoles. Sci China Chem 2022;65:1929-37.
131. Ma C, Jiang F, Sheng FT, Jiao Y, Mei GJ, Shi F. Design and catalytic asymmetric construction of axially chiral 3, 3'-bisindole skeletons. Angew Chem Int Ed Engl 2019;58:3014-20.
132. Tian M, Bai D, Zheng G, Chang J, Li X. Rh(III)-catalyzed asymmetric synthesis of axially chiral biindolyls by merging C-H activation and nucleophilic cyclization. J Am Chem Soc 2019;141:9527-32.
133. Sheng F, Li Z, Zhang Y, et al. Atroposelective synthesis of 3, 3’-bisindoles bearing axial and central chirality: using isatin-derived imines as electrophiles. Chin J Chem 2020;38:583-9.
134. Chen KW, Wang ZS, Wu P, et al. Catalytic asymmetric synthesis of 3, 3'-bisindoles bearing single axial chirality. J Org Chem 2020;85:10152-66.
135. Sheng F, Yang S, Wu S, Zhang Y, Shi F. Catalytic asymmetric synthesis of axially chiral 3, 3'-bisindoles by direct coupling of indole rings. Chin J Chem 2022;40:2151-60.
136. He X, Zhao H, Song X, Jiang B, Du W, Chen Y. Asymmetric Barton-Zard reaction to access 3-pyrrole-containing axially chiral skeletons. ACS Catal 2019;9:4374-81.
137. Wu P, Yu L, Gao C, et al. Design and synthesis of axially chiral aryl-pyrroloindoles via the strategy of organocatalytic asymmetric (2+3) cyclization. Fund Res 2023;Epub ahead of print.
138. Xia Y, Liu M, Qian C, Li P, Dong M, Li W. Asymmetric organocatalytic (3+2) annulation of propargylic alcohols with indolylnaphthalenols: synergistic construction of axial and central chirality. Org Chem Front 2022;10:30-4.
139. Wang F, Jing J, Zhao Y, et al. Rhodium-catalyzed C-H activation-based construction of axially and centrally chiral indenes through two discrete insertions. Angew Chem Int Ed Engl 2021;60:16628-33.
140. Mi R, Chen H, Zhou X, et al. Rhodium-catalyzed atroposelective access to axially chiral olefins via C-H bond activation and directing group migration. Angew Chem Int Ed Engl 2022;61:e202111860.
141. Qin J, Zhou T, Zhou TP, et al. Catalytic atroposelective electrophilic amination of indoles. Angew Chem Int Ed Engl 2022;61:e202205159.
142. Ji D, Jing J, Wang Y, et al. Palladium-catalyzed asymmetric hydrophosphination of internal alkynes: atroposelective access to phosphine-functionalized olefins. Chem 2022;8:3346-62.
143. Wang HQ, Wu SF, Yang JR, Zhang YC, Shi F. Design and organocatalytic asymmetric synthesis of indolyl-pyrroloindoles bearing both axial and central chirality. J Org Chem 2022;Online ahead of print.
145. Terada M. Binaphthol-derived phosphoric acid as a versatile catalyst for enantioselective carbon-carbon bond forming reactions. Chem Commun 2008:4097-112.
146. Terada M. Chiral phosphoric acids as versatile catalysts for enantioselective transformations. Synthesis 2010;2010:1929-82.
147. Yu J, Shi F, Gong LZ. Brønsted-acid-catalyzed asymmetric multicomponent reactions for the facile synthesis of highly enantioenriched structurally diverse nitrogenous heterocycles. Acc Chem Res 2011;44:1156-71.
148. Parmar D, Sugiono E, Raja S, Rueping M. Complete field guide to asymmetric BINOL-phosphate derived Brønsted acid and metal catalysis: history and classification by mode of activation; Brønsted acidity, hydrogen bonding, ion pairing, and metal phosphates. Chem Rev 2014;114:9047-153.
149. Xia ZL, Xu-Xu QF, Zheng C, You SL. Chiral phosphoric acid-catalyzed asymmetric dearomatization reactions. Chem Soc Rev 2020;49:286-300.
150. Li S, Xiang S, Tan B. Chiral phosphoric acid creates promising opportunities for enantioselective photoredox catalysis. Chin J Chem 2020;38:213-4.
151. Lin X, Wang L, Han Z, Chen Z. Chiral spirocyclic phosphoric acids and their growing applications. Chin J Chem 2021;39:802-24.
152. Cambridge Crystallographic Data Centre. CCDC 2227139 for (Sa)-3aa. Available from: https://www.ccdc.cam.ac.uk/.[Last accessed on 1 Feb 2023].
Cite This Article
How to Cite
Wu, P.; Yan, X. Y.; Jiang, S.; Lu, Y. N.; Tan, W.; Shi, F. Organocatalytic Nazarov-type cyclization of 3-alkynyl-2-indolylmethanols: construction of axially chiral cyclopenta[b]indole scaffolds. Chem. Synth. 2023, 3, 6. http://dx.doi.org/10.20517/cs.2022.42
Download Citation
Export Citation File:
Type of Import
Tips on Downloading Citation
Citation Manager File Format
Type of Import
Direct Import: When the Direct Import option is selected (the default state), a dialogue box will give you the option to Save or Open the downloaded citation data. Choosing Open will either launch your citation manager or give you a choice of applications with which to use the metadata. The Save option saves the file locally for later use.
Indirect Import: When the Indirect Import option is selected, the metadata is displayed and may be copied and pasted as needed.
About This Article
Special Issue
Copyright
Author Biographies
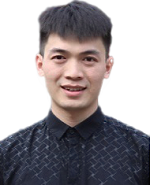
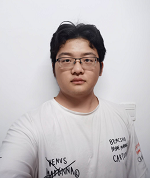
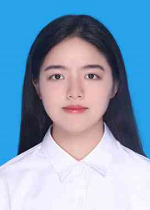
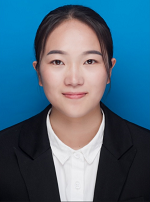
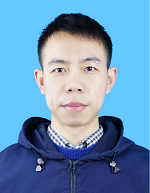
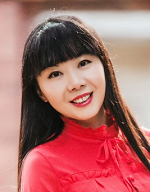
Comments
Comments must be written in English. Spam, offensive content, impersonation, and private information will not be permitted. If any comment is reported and identified as inappropriate content by OAE staff, the comment will be removed without notice. If you have any queries or need any help, please contact us at [email protected].