Towards wearable sensing-based precise and rapid responding system for the early detection of future pandemic
Abstract
During the past three years, tremendous efforts have been made to tackle the Coronavirus Disease 2019
Keywords
INTRODUCTION
The COVID-19 pandemic has had a profound and far-reaching impact on the world since it emerged in 2019. According to the latest statistics from the World Health Organization (WHO) on 9 August, 2023[1], over 769 million confirmed cases of COVID-19 and approximately 6.9 million fatalities have been reported. The outbreak has threatened global public health and the economy over the past three years. There have been some changes in public perception and policy, such as the WHO's acknowledgment that a zero-COVID strategy is unsustainable[2] and the lifting of compulsory quarantine. Nonetheless, the emergence of viral varieties and the long-term effects of infections[3] show that it requires continuous attention and management. Particularly in China, the COVID-19 resurgence has meant three-quarters of Chinese people who were not infected in the initial wave were infected in subsequent waves[4].
The severity of the crises resulting from the COVID-19 outbreak and its resurgence highlights the urgent need for swift responses and anticipatory solutions in combating highly contagious diseases. Initiatives such as the proactive global eco-social pandemic preparedness system[5] and a technical framework[6] for reacting to COVID-19 have been presented. Though some researchers have developed models to understand the current and future pandemics[7], society still faces grand challenges in predicting the occurrence of future pandemics, and how likely they will affect the health of human beings.
For successful early prediction and management of pandemics, the implementation of self-reporting of symptoms and routine monitoring has been a key tenant. To promote adherence and ensure wide adoption of a monitoring approach, there is a significant demand for straightforward and portable tracking technologies capable of monitoring vital physiological, biochemical, molecular health metrics and disease indicators. These requirements can be effectively addressed by wearable devices. Wearables are being increasingly integrated into clinical practice to support health assessment and early detection, becoming more prevalent not only for individual and home use, but also in clinical settings[8]. Therefore, it is essential to incorporate Internet of Things (IoT) assisted wearable health sensors into the response and management systems to identify anomalies, detect emerging patterns and predict the likelihood and localization of a disease outbreak before it becomes widespread.
Several comprehensive reviews have been conducted regarding wearable technologies on COVID-19. Many of them lack the perspective of molecular testing supported by wearables[9-13], which plays a crucial role in achieving early detection, especially if it could detect low virus loads before infected individuals become contagious. Our study covers wearable molecular testing to support combating the current COVID-19 circumstance and possible future pandemics. Furthermore, our study emphasizes a wearable sensing-based precise and rapid responding system, leveraging state-of-the-art GPT algorithms to evaluate multimodal data, especially from wearables, to provide personalized suggestions and prompt responses. Existing studies either primarily focus on the early detection, assessment and monitoring of COVID-19[9,10], or on managing long COVID conditions[11]. The review from Ding et al. categorizes enabling wearable technologies by symptoms and telehealth technologies for the remote monitoring and diagnosis of COVID-19[12]. Adans-Dester et al. discusses the significance of mHealth technologies and their integration to mitigate the effects of the COVID-19 pandemic[13]. Compared with the aforementioned works, the present study not only provides a summary of wearable technology applications in the early detection, diagnosis and monitoring of COVID-19, but also highlights their role in managing long COVID. Our proposed response system involves the emerging GPT algorithms and several mHealth technologies for addressing COVID-19 and future pandemics, and can be extended to tackle other diseases with minor modifications.
In the envisioned system, wearable devices play a crucial role in tracking physiological health data and potentially even detecting related molecular biomarkers associated with a disease. Furthermore, the suggested system will deliver remote healthcare services and facilitate population monitoring, particularly for high-risk groups. By reviewing the applications of mentioned technologies, we aim to shed light on how they can assist in the early detection and monitoring of outbreaks. Lastly, the aforementioned wearable sensing-based responding system is proposed as a promising solution to address the prevailing challenges encountered in pandemic prevention, early detection and effective management, ultimately enhancing our ability to be better prepared and to respond effectively to such crises in the future.
WEARABLE TECHNOLOGIES FOR COVID-19
As stated in several studies[14-18], fever, respiratory abnormalities, fatigue, muscle pain, headache, and other cardiac complications are common in pre-symptomatic cases, symptomatic cases, and post-COVID-19 patients. Wearable devices, aided by mHealth technologies, are critical technologies necessary to continuously track and monitor diseases through physiological, physical, and biochemical sensing. The COVID-19 pandemic has further advanced this field. Being able to collect relevant physiological data and self-reported symptoms, wearable devices were suggested to be included in COVID-19 management for detecting infectious cases, reducing disease transmission, facilitating early intervention and monitoring long-term effects. Several wearables for COVID-19 are summarized in Table 1.
A summary of wearable technologies for monitoring of COVID patients
Clinical symptoms | Measurements | Exemplary wearable products/prototypes |
Fever | Temperature | Finger ring (Oura[19]), wrist band/watch (Apple[20], GOQii[21]), armband (Everion[22]) |
Respiratory symptoms (i.e., dry cough, shortness of breath) | Cough signal | Face mask[23], neck patch[24], shirt[25], smartphone[26], microphone[27] |
RR | Face mask[23,28], patch[24,29,30], wrist watch/band (WHOOP[31]), patch (BioButton[32]), stethoscope[33], shirt[34], microphone[27] | |
Lung sound | Shirt[25], stethoscope patch[33,35] | |
SpO2 | Wrist band/watch (Samsung[36]), shirt (Hexoskin[37]), fingernail[38], earbud[27,39], patch[40] | |
Cardiac symptoms (i.e., arrhythmia, myocardial injury, chest pain) | ECG/HR | Shirt/vest[41], flexible patch[42,43],fingernail[44] |
Heart sound | Shirt/vest[45,46], smartphone[47], stethoscope patch[33] | |
Cuffless BP | Wrist watch/band (Biobeat[48]), finger clip (Valencell[49]), fingernail[50], flexible patch[51], tattoos[52], earbud[53] | |
Fatigue | Fatigue level | Patch[54], glasses[55], shirt[37] |
EMG, EEG | Shirt[25], earbud[56], flexible path[42,43] | |
Pain (i.e., body aches, headache) | ||
Sleep disturbance | ECG/HR, PSG, EOG, etc. | Shirt[57], wrist-worn Actigraphs[58], WAKE Earpiece[59], ring[60] |
Wearables for detecting early signs of COVID-19
COVID-19 patients may be contagious 48 h before starting to experience symptoms, i.e. while being pre-symptomatic or asymptomatic[61]. Laboratory data from WHO demonstrated that the highest level of infectivity occurs just before symptom manifestation and during the initial phases of the disease[62]. Consequently, timely detection of early signs of COVID-19 may not be sufficiently rapid to identify infected individuals before they become contagious. However, early identification of the infection could help in immediate intervention and controlling the further spread of the disease, particularly during the incubation stage. Figure 1 summarizes a wide range of wearables, including smartwatches, finger rings, face masks, earbuds, wristbands, armbands, smart textiles, and patches, for evaluating the early signs or clinical symptomology of COVID-19.
Some findings demonstrated that temperature could be used as a superior indicator for COVID-19 detection before individuals become symptomatic[19,34]. Fever is one of the most common symptoms observed in over 50% of infected patients[63]. Commercialized wearables such as wristwatches/bands (GOQii, GOQii Technologies Pvt Ltd, United States)[21] and armbands (Everion, Biofourmis, Switzerland)[22] can measure temperature continuously. Studies conducted by the University of California using Oura Ring (ura Health Oy, Finland) suggest that data changes 76% of the time before users feel symptoms of COVID-19[19,64].
Abnormalities in respiratory symptoms, such as dry cough and shortness of breath, have been demonstrated to be one of the earliest signs of COVID-19 infection[65]. To detect variations in respiratory activities, several innovative wearable approaches based on chest/throat vibrations[24,29], air pressure/vibrations[23], acoustic sensing[25,35], ultrasound[66], or others[30] have been developed. One such example is the Rogers team's[24] soft, skin-integrated, high-bandwidth, accelerometer-based monitoring patch attached at the base of the neck, offering continuous readings of respiratory rate (RR), coughing, body temperature, etc. Several researchers integrated sensors on face masks for detecting air pressure/vibrations for respiratory monitoring[23,28]. Additionally, nasal respiratory sensors are widely explored[30,67], one of which is capable of simultaneously capturing multiple self-calibrated breathing parameters (such as respiration depth, apnea-hypopnea index, and peak expiratory flow) for evaluation[30]. Respiratory activities including RR and cough patterns can be measured and identified by utilizing a smartphone[26], stethoscope[35], or an acoustic sensor[25]. Several respiratory monitoring systems, such as BioButton (BioIntelliSense, Inc., Australia)[32], have been commercialized. Additionally, blood oxygen saturation level (SpO2) is an important indicator for early warning, triage, and monitoring of COVID-19 patients[68]. There are numerous pulse oximetry devices based on optical sensing available in the market, especially worn on the wrist and fingertip from companies such as Apple (Apple Inc., United States), Huawei (Huawei Technologies Co., Ltd., China), and Samsung (Samsung Electronics Co., Ltd., South Korea)[36]. Even though the technology is well-established, various studies continue to investigate SpO2 measurements via flexible patches, earbuds, and fingernails[38-40].
According to the report from Columbia University’s Division of Cardiology in New York, the virus injures the heart, probably by either attacking it directly or causing a cytokine storm that leads to myocyte apoptosis[69]. Cardiac symptoms can be the first sign of COVID-19, with reports from China documenting patients who experience palpitations and chest pain without the typical fever and cough[17]. Therefore, the monitoring of certain cardiovascular indicators, such as electrocardiogram (ECG), heart rate (HR), and blood pressure (BP), is of critical importance. To assess early cardiovascular involvement in COVID-19 patients, ECG is a widely used clinical method. Various abnormalities in ECG such as ST- and T-wave changes, arrhythmia and myocardial infarction have been reported in COVID-19[70]. One study presented a cognitive dynamic system (CDS)-based framework, which is able to automatically identify people having arrhythmia from single-lead ECG traces[71]. Some special designs such as non-contact wearable wireless ECG systems based on capacitive-coupled active dry electrodes are also proposed[72,73]. ECG wristbands and chest patches are frequently used for wearable ECG monitoring, most of which are commercially available and have received FDA certification. However, both patch electrodes and wristwatches/bands are mostly single lead systems, which may not be enough to detect cardiac abnormalities, especially myocardial infarction. Many investigations have been carried out to produce multiple-lead ECG monitoring[41,42]. One such example, using a graphene/PDMS dry electrode material, has recently attracted attention as it is stable, washable, flexible, and reusable. The integrated electrode provides a novel strategy for wearable 12-lead ECG monitoring and could be extended to professional EEG and EMG monitoring[42].
COVID-19 can cause increases or irregularities in HR in response to fever or inflammation[74]. The smartwatch is already being used for HR monitoring. One study analyzed the resting HR data collected from 1,265 individuals, which could distinguish between COVID-19-positive and negative cases as early as 10 and 5 days prior to a diagnostic test[75].
Even though fatigue is a less well-known symptom of COVID-19, it is one of the early signs of the disease and more common than the “classic” symptoms of cough and fever[15]. Additionally, pain is also one of the early signs of coronavirus infection, especially headaches and muscle aches[16]. There are various products or prototypes in the form of glasses[55], patches[54], and earbuds[56] to evaluate fatigue levels and pain perception. Particularly, Northwestern University researchers developed a soft, flexible, postage-stamp-sized wearable device that comfortably adheres to the upper chest to evaluate vocal fatigue and provide real-time feedback[54]. These technologies could be potentially useful for detection of early signs of COVID-19. Several selected wearable devices with potential applications in COVID-19 and the representative results are presented in Figure 2.
Figure 2. Selected wearable devices with potential applications in COVID-19 and the representative results. (A) Oura smartrings, temperature, HR, HRV and RR were examined prior to and during the reported symptom window, and it was found that many showed similar patterns[64,76]; (B) Bioimpedance ring, SBP (blue line) and DBP (orange line) estimations with bioimpedance ring sensor in reference to gold-standard Finapres finger BP cuff measurements (gray circles)[77]; (C) A suprasternal notch-mounted sensing patch for tracking of cardiopulmonary activity during cycling, comparisons of RR and HR determined by the dual-sensing (blue square), single-sensing (red circle) and reference devices (green triangle)[24].
Despite the fact that accurate and fast early detection of COVID-19 by molecular testing has not yet been accomplished, there has been considerable research into prediction and early detection of the disease using mathematical and computational models with AI assistance[78,79]. Some studies and products use information from abnormalities/variations in physiological features to identify COVID-19 prior to the symptom onset or in asymptomatic cases. These features include analysis of RR[65] and wristwatch data (HR, daily step count, or sleep duration)[80]. Available products include the European CE-marked Empatica smartwatch (Empatica Inc., Italy)[81] and the FDA-approved armband Tiger Tech COVID PlusTM Monitor (Tiger Tech Solutions, Inc., United States)[82,83].
Wearables for molecular detection
Some existing methods documented that they could identify pre-symptomatic or asymptomatic cases, while the concern is that even without COVID symptoms, individuals may be infectious, making immediate detection and confirmation of COVID-19 before transmission extremely difficult. In light of this, a study conducted by a team from Harvard University and MIT developed a synthetic biology sensor (lyophilized CRISPR sensor) placed into a face mask. Thus, the sensors are able to detect SARS-CoV-2 noninvasively at room temperature in less than 90 min. The face mask sensor demonstrates a detection limit of 500 copies (17aM) of SARS-CoV-2 in vitro transcribed (IVT) RNA, equivalent to the detection limit of WHO-endorsed standard laboratory-based RT-PCR assays, while also exhibiting no cross-reactivity to RNA from other commonly circulating human coronavirus strains (HCoVs) [Figure 3]. These sensors can be integrated into garments with sensing electronics and wireless networking capabilities for real-time dynamic monitoring of target exposure[84,85]. Though the sensitivity and selectivity are highlighted, the results might not be rapid enough to prevent transmission, typically before they engage in crowd gatherings or other high-risk social activities. Another work from Cao’s team at Peking University[86] could predict key mutations by studying antibodies from people who had recovered from the earlier variants.
Figure 3. A face-mask-integrated SARS-CoV-2 wearable diagnostic system. (A) Photographs of the SARS-CoV-2 sensor integrated into a face mask; (B) Breath emission simulator setup; (C) Sensitivity of the A-version face mask sensors at various inputs on the sensor zone of IVT-generated SARS-CoV-2 S-gene RNA; (D) Representative images of lateral flow assay (LFA) outputs from the sensitivity measurements; (E) Specificity demonstration of the sensors shows no cross-reactivity with IVT RNA from other commonly circulating HCoVs; (F) Representative images of LFA outputs from the specificity measurements. Cited from Nguyen et al.[85]
Although wearables for continuous physiological and physical sensing aided by AI have shown promising advancements in detecting early signs of COVID-19 or even identifying the virus before symptoms appear, they are limited by the uncertainty of infectiousness. To truly achieve early detection, there is a need for further exploration and integration of wearable devices with highly sensitive biochemical sensors capable of detecting low virus loads even before infected individuals become contagious. These sensors should be developed and incorporated into a wearable format to enhance their usability and practicality for real-time monitoring and early identification of COVID-19 cases before the subjects become contagious.
Wearables for monitoring of COVID patients
Those who received a positive COVID-19 test were more likely to have similar symptoms as pre-COVID cases, whereby their health status can be tracked and monitored continuously and remotely by the summarized wearable technologies above.
High blood pressure (BP) has been identified as one of the most common comorbidities and risk factors for severity and adverse outcomes in COVID-19 patients[87]. Another large observational study reported a small but consequential rise in average BP among US adults during the COVID-19 pandemic, which could significantly increase the number of strokes and heart attacks in the general population[88]. Cuffless BP monitoring devices are crucial for BP monitoring because they can readily provide continuous, real-time monitoring. Several commercialized cuffless BP wearable products are available, such as Biobeat (Biobeat Technologies, Israel)[48] and Valencell (Valencell, Inc., United States)[49], worn on the wrist and fingertip, respectively, for beat-to-beat BP monitoring. Other than these, some researchers explored unusual sites such as in the ear[53], finger proximal phalanx[77] and fingernail[50], as well as new approaches such as multiwavelength photoplethysmography (PPG)[89]. In order to improve the wearing conformability and unobtrusiveness, several biocompatible sensors such as silk nanofibrous iontronic pressure sensors[51] and graphene bioimpedance tattoos[52] are in development for skin-friendly longer-term BP measurement.
Difficulty breathing, inability to wake or stay awake, and cognitive dysfunction are also reported as severe situations in COVID patients[14]. Some studies investigated the associations between cognitive dysfunction and physiological signals such as HR, temperature, and skin conductance and behavioral signals, which can be monitored using embedded sensors from wearables like the Microsoft Band 2 smart band (Microsoft Corporation, United States)[58] or from simpler wireless peripherals such as PPG devices[90]. With the purpose of facilitating symptom monitoring and sleep disorder identification, a wearable shirt was devised[57]. Another approach used a wrist-worn Actigraph (ActiGraph, LLC., United States)[58], which can track both waking and sleeping hours. The behind-the-ear wearable technology by Pham et al. may concurrently detect sleep and fatigue[59]. All of them can be potentially used in COVID scenarios.
Infected patients typically manifest a combination of at least two symptoms instead of a single symptom[63]. However, the need to wear several sensors to obtain multiple related signals is not an ideal approach as it will lead to poor compliance and has the potential for compounded readout errors due to errors in sensor placement and misuse by the end-user. Alternatively, it may be possible to extend sensor or system functionality by innovative multimodal design to obtain multiple signals simultaneously. Furthermore, with advantages in novel material and fabrication technologies, developing flexible, stretchable, self-adhesive, lightweight wearable sensors is gaining increased attention [Figure 4]. Multimodal sensor design can extract more sophisticated information, while flexible sensing is advantageous for achieving precise, unobtrusive, long-term monitoring. This integration enables the detection of unusual disease patterns or clusters of symptoms, thereby providing early warning signals for potential pandemics.
Figure 4. (A) A wide-bandwidth nanocomposite-sensor integrated smart mask for tracking multiphase respiratory activities[23]; (B) A smart ultrathin pressure sensor-based face mask for breath monitoring[28]; (C) An electronic tattoo for sensing multiple signals[43]; (D) Epidermal electronics for respiration monitoring[67]; (E) A wearable cardiac ultrasound imager[91].
Wearables for long COVID
The WHO reported that a significant proportion of individuals infected with COVID-19, ranging from 10% to 20%, may continue to develop long-COVID sequelae[18]. Since COVID is a multi-organ disease, there is a growing awareness of how to manage these ongoing health issues. Apart from the commonly observed symptoms such as fatigue and cough[92], emerging evidence from various reports highlights an increased incidence of psychological and psychiatric disorders among individuals who were infected. These include sleep disturbances both during and after the pandemic[93-95]. It is crucial to effectively manage these wide-ranging health consequences associated with COVID-19, extending beyond the acute phase of the illness.
With the rise of wearables, it is important to evaluate the long-term effects of COVID-19, particularly on sleep patterns, by using wearables. Some researchers have utilized PPG-based wearable devices to analyze sleep phases, and certain biometrics are highly related and can reflect sleep patterns[96]. Furthermore, machine learning algorithms have been developed using the relationship between biometrics (e.g., HR and HRV) and sleep cycles to define sleep phases[97,98]. Mekhael et al. used wearable PPG-based wristbands to detect RR and SpO2 to assess the long-COVID effects on sleep patterns[99]. The Oura ring that has been evaluated against polysomnography (PSG), the gold standard test used in sleep labs, can also be used to monitor post-COVID insomnia[60]. Moreover, the risk of long COVID may lead to life-threatening conditions. For example, a significant drop in SpO2 levels (less than 95%) may cause brain damage, heart failure, or even sudden death[11], which can be monitored by wearable devices.
Long COVID-19 encompasses various unknown factors and the management and treatment approaches for this condition are still in their early stages[100,101]. Although several studies acknowledged that wearables could be a viable solution for recognizing long COVID and emerging infections, long COVID involves a spectrum of cardio-neuro effects, such as brain fog, insomnia, dizziness, and blood lipid abnormalities[102]. Therefore, wearable devices alone are insufficient to identify and manage long COVID patients. Rather, they should be supplemented with imaging tests (chest X-rays, CT scans), pulmonary function tests, hematological assessments, etc.
Personalized AI Doctor (Doctor PAI)
The widespread use of AI has been facilitated by the abundance of complex big data in the healthcare industry[103], particularly in the context of the ongoing COVID-19 pandemic and future outbreaks. Numerous studies have demonstrated that AI is capable of performing or even outperforming humans in several healthcare-related tasks, such as analyzing vast amounts of medical data to provide diagnoses and identifying patterns that may not be immediately apparent to human healthcare professionals[104]. It is anticipated that AI will eventually change the remote healthcare domain with applications in teleconsultation, rehabilitation, health management and other areas as a fundamental tool driving the advancement of precision medicine. The application of AI is suggested for the fight against COVID-19 and can be used for early warning systems, ongoing monitoring, diagnosis and prognosis, therapy, and even drug design, as well as control over society and individuals via regulations. Several AI-based models, such as BlueDot[105] and HealthMap[106], have been developed and were shown to be capable of predicting infectious pandemic diseases. Moreover, Bluedot predicted the COVID-19 threat before WHO officially recognized it at the end of December 2019[107].
Recently, GPT described algorithms, such as ChatGPT, have attracted much interest in clinical medicine[108-110]. ChatGPT was able to manage complicated medical and clinical information[111], and passed the US Medical Licensing Test without clinician input[112]. When ChatGPT was asked what specific information would enable early diagnosis, control and management of COVID-19, the responses clearly mentioned that the integration of health data collected from wearables can provide additional insights for personalized preventive measures. Furthermore, to answer the question of “What kind of system would you suggest for the early detection of future pandemics?”, ChatGPT proposed to implement a comprehensive system encompassing a global surveillance network, advanced data analysis, integrated healthcare systems, etc., with a focus on global collaboration, information sharing, advanced analytics and early intervention. With such a system, it would be feasible to identify and respond to infectious diseases promptly, implement appropriate control measures, and effectively manage the initial phase of transmission. However, it should be noted that the provided suggestion lacks specific technical details and operational guidelines. When asked the technical question regarding the role of physiological and molecular sensing, ChatGPT recognizes the crucial roles of physiological and molecular sensing in the forecasting and timely identification of future pandemics, especially in providing valuable contributions in disease biomarker identification, rapid diagnosis, wearable devices, syndromic surveillance, remote monitoring and telemedicine, early warning systems, among others, as outlined in Appendix Table 1 as an Appendix. The full responses emphasized that by processing comprehensive and up-to-date information on different aspects such as symptoms, contact tracing, and integrating wearable health data, they could provide valuable data for surveillance, diagnostics and public health interventions, ultimately helping to mitigate the impact of pandemics on global health. These insights align closely with the proposed responding system we have introduced.
It is expected that the infection risks can be reduced by the development of a Doctor PAI system that integrates and evaluates multimodal data from wearables, medical imaging, molecular tests and contact tracing, leveraging a GPT model to provide personalized and fast responses to users' health-related inquiries. Upon utilizing the proposed Doctor PAI system, the data is uploaded to a cloud-based storage bucket and processed by the Doctor PAI, which utilizes both GPT and AssemblyAI to interpret textual and voice messages. The pre-trained GPT model is stored in a Lambda function on the cloud, enabling it to handle user queries and retrieve relevant information from the database. The system is designed to be scalable and capable of managing large volumes of data while concurrently ensuring user privacy and data security. By deploying on wearables, smartphones and other platforms, the benefits of Doctor PAI for general populations include professional medical advice, AI-assisted living, and homecare, allowing for tele-consultations, tele-monitoring, tele-diagnosis and even tele-intervention as depicted in Figure 5. Furthermore, Doctor PAI might arm caregivers with powerful tools for a more thorough evaluation, a precise and prompt diagnosis, and specialized COVID patient care, simultaneously alleviating the workload of healthcare professionals and enhancing overall medical efficiency.
Figure 5. The overall design of Doctor PAI and schematic representation of how to utilize Doctor PAI. Textual or voice messages of inquiries, symptoms, personal information and medical history from the user (or from the medical institution) can be interpreted by Doctor PAI. These data are subsequently analyzed by current GPT technologies to obtain potential diagnoses. Together with physiological health data collected from wearables, available medical imaging, DNA testing and blood testing data from medical institutions, Doctor PAI integrates and evaluates the multimodal health data to deliver personalized and prompt responses.
Smart conversational agents (chatbots), including ChatGPT, are currently being widely debated due to both the advantages highlighted above and the disadvantages described next. Indeed, utilizing AI in clinical diagnosis in real-world implementation presents some pitfalls. One such pitfall is that the GPT models are limited by training on a general information database instead of a specific medical database. ChatGPT does not source information in the traditional sense and cannot evaluate its output’s reliability. Instead, the model relies on the likelihood of phrases and sentences going together rather than by reference to specific knowledge bases. This limitation may result in inaccuracies and pose a strong safety concern[113]. Correspondingly, AI cannot serve as a substitute for healthcare providers due to the inherent risk of outdated or biased information when information sources are not consistently updated or not heterogeneous. The consistent use of ChatGPT for diagnosis by physicians may lead to a decline in their diagnostic capability and overreliance on technology[108]. Furthermore, a significant concern arises from the absence of accountability regarding medical accidents and associated errors. This gap can be exploited by persons with malicious intent to develop substandard products or to acquire patients' personal information. Notwithstanding its inherent challenges, GPT technology showcases numerous advantages and possesses significant potential applications. If employed appropriately, in the future, this may represent a groundbreaking and innovative approach that has far-reaching implications for traditional medical systems, expanding its potential applications to various diseases beyond COVID-19.
WEARABLE-BASED PRECISE AND RAPID RESPONDING SYSTEM
According to the WHO, the early infectious period of COVID-19 without recognizable symptoms is around 2-3 days[62]. If the signs/signals could be caught in that time window together with immediate quarantine, it would significantly reduce disease transmission. For this, a wearable-based precise and rapid responding system is proposed [Figure 6]. It is easily accessible to the public, with the benefits of early detection and long COVID monitoring for better management of future pandemics or long COVID.
In the proposed system, the use of wearables would enable real-time physiological signals/metrics and biochemical markers monitoring[81,84,114]. With continued innovation, several wearable-based mHealth technologies could be developed with contact tracing applications[115-118]. This is considered to be the first and most effective step to prevent an outbreak when the medical resources are unavailable for immediate utilization[119], allowing for the tracing of people and assessing the possibility of infection via contact with other suspected individuals or individuals who tested positive. Users can conveniently access their health data through wearables or mobile devices wirelessly. Furthermore, a feasible real-time wearable system with a control panel and a vital-sign data display can be remotely operated by authorized healthcare professionals. It is important to strengthen trust and improve the accuracy of remote monitoring through collaborative efforts with clinicians, such as proposing clinician dashboards in wearable systems[120]. In addition to the above-mentioned technologies, the Centers for Disease Control and Prevention (CDC) launched the National Wastewater Surveillance System (NWSS) in September 2020 to provide an early warning of COVID-19’s spread in communities[121]. States and municipalities such as Hong Kong monitor wastewater effluent to assess viral emergence and resistance[122]. Due to the difficulties in predicting outbreaks and capturing asymptomatic carriers in a timely manner, sewage or wastewater monitoring can be used to trace and track the presence of SARS-CoV-2, and screen entire communities, becoming a valuable indirect epidemiological prediction tool for COVID or other pandemic viruses. Several methods including polymerase chain reaction (PCR), plaque-forming test and laboratory-on-a chip can be used for virus quantification, and can provide an alert if viral particles rise above recommended thresholds[123,124]. The implemented AI technology would help with the integration of collected information and aid in the identification of patterns and anomalies for early detection of COVID, allowing for quicker action and containing the infection before it spreads at an alarming rate. If warning signs occur, the AI-assisted system would suggest that individuals seek molecular testing immediately for clinical diagnosis. The system would recommend suitable and effective isolation actions such as self-isolation at home with physiological and symptoms monitoring, centralized quarantine, or even partial lockdown to break the transmission chain.
Using the aforementioned wearable technologies, mHealth, and telemedicine combined with AI for data fusion and decision making, it is possible to control the spread of the disease in its earliest phase and obtain immediate medical intervention in case of clinical deterioration. It is also possible to suggest whether people need additional medical examinations (i.e., imaging), decide whether to keep people isolated or quarantined, and maintain frequent and ongoing monitoring of patients who are experiencing COVID or long COVID. Moreover, a published recommendation advocates for the utilization of wearable-based mHealth technologies in the closed-loop management of acute cardiovascular disease (CVD) patients during the COVID-19 pandemic[125]. Another study proposed a wearable telehealth system for monitoring COVID-19 and chronic diseases[114]. Given the resemblance in devices and technologies employed, with minor adaptations, the proposed responding system shows potential for extending its applications beyond COVID-19, specifically in addressing cardiovascular diseases in non-pandemic situations. The system can seamlessly transition to effectively respond to pandemic situations during outbreaks, and may help to optimize the efficiency and effectiveness of managing urgent patient care and reducing infection risk.
Furthermore, the widespread adoption of fluid biomarker testing, including PCR, has become standard practice to ensure safe transit, such as airline travel, especially during the peak periods of a pandemic. However, a significant drawback of this approach is the time required to receive test results. This delay can potentially yield incorrect negative results if an individual becomes infected during the interim period between testing and result delivery, consequently enabling viral transmission. An additional promising application of the proposed system is its integration with digital passports. It can help relevant sectors to validate passengers’ health documents and use their real-time electronic health data in a safe and secure way to facilitate real-time infection monitoring. A noteworthy milestone occurred on 1 July 2023, when WHO took up the European Union (EU) system of digital COVID-19 certification to establish a global system that will help protect citizens across the world from on-going and future health threats, including pandemics[126,127]. Overall, the integration of these digital approaches is a transformative development that offers a wide range of benefits, including real-time infection monitoring, rapid contact tracing, streamlined travel processes, safe free movement, enhanced public health surveillance, and optimized resource allocation. The adoption of such digital approaches necessitates careful consideration of legal and validation steps, which involves close collaboration among governments, regulatory bodies, healthcare providers, technology developers, and researchers[128,129].
CHALLENGES AND PERSPECTIVES ON PREDICTION OF FUTURE PANDEMICS
This paper provides an overview of wearables and their potential applications in combatting COVID-19, including early prediction, physiological monitoring, molecular detection, and long COVID patient care. We suggest a wearable-based precise and rapid response system and highlight the capabilities of wearable technology with contact tracing and AI support. Despite the immense potential of wearables, there are numerous obstacles that prevent their widespread usage. In this section, key challenges with the use of wearables are identified, and promising directions are suggested for a better population and patient health response to the COVID-19 pandemic and to possible future pandemic emergencies.
(1) To the best of our knowledge, there are no wearables available for rapid molecular detection for the early detection of COVID-19. Developing highly sensitive molecular detection wearables capable of detecting the virus in a low load before the patients become contagious could effectively cut the transmission chain to avoid disease spread.
(2) Multiple sensors for obtaining several related signals at the same time can result in discomfort, higher costs, and compliance issues. Innovations in multimodal sensor design to continuously monitor large-scale data streams from multiple sources may provide more detailed information than any single sensor.
(3) With advanced material and fabrication technology, flexible and stretchable sensing-based wearable devices for COVID have been extensively investigated. Even if it could be worn comfortably for unobtrusive monitoring, there still exist issues such as the trade-off between thin-film design and power supply, sliding during motion, irritability, and long-term adherence.
(4) Combining biochemical sensors to gather molecular information and physical sensors to monitor physiological signals is a potential direction for the next generation of wearables. Integrated sensors on wearables may be employed as a substitute method for predicting COVID progression, early detection, monitoring health status, and diagnosis of the disease and its long COVID impacts. Though the sensitivity and selectivity are highlighted, there are still issues in accuracy and speed.
(5) It is worth exploring wearable technologies utilizing 2D images or high dimension data, allowing for continuous and real-time image assessment, such as cardiac function assessment[91], which could be beneficial for patients experiencing severe symptoms or long COVID effects. Further work is required to increase spatial resolution, create novel reconstruction algorithms, and miniaturize the entire system, particularly the data processing and power supply backend.
(6) Other technical and usage challenges are inevitable in the development of wearables, including real-time data processing, lack of wide acceptance by clinical professionals[130], power consumption and cost-effectiveness, and user comfort (especially for long-term monitoring), all of which continue to limit the effective adoption and uptake of wearable technology.
(7) Compared with manual contact tracing, developing wearable-based mHealth contact tracing with symptom tracing could not only identify individuals who were in contact with a COVID-positive patient, but also identify if contacts were made with pre-symptomatic or asymptomatic patients. However, accuracy, response time, and privacy issues are areas that need further addressing with these approaches. Additionally, a contact-tracing application with the potential to be widely adopted by a free market must consider a fair trade-off between several design constraints, including trust constraints, reliable range constraints, and low/ultra-low energy consumption constraints[131].
(8) It is challenging to develop effective methodologies to integrate and evaluate complex, multimodal healthcare data such as wearable sensing, biomarkers and imaging information with GPT technologies to enable tele-consultations, tele-monitoring, tele-diagnosis, and even tele-intervention to receive specialized COVID patient care from hospitals to homes/individuals.
(9) The benefits for general populations include professional medical advice, AI-assisted living, and homecare provided by virtual doctors who enable tele-consultations, tele-monitoring, tele-diagnosis, and even tele-intervention. Furthermore, it might arm caregivers with powerful tools for a more thorough evaluation, a precise and prompt diagnosis, and specialized COVID patient care.
(10) It is worth conducting large-scale feasibility and validation studies on the use of wearables to provide similar information as those from clinical tests such as pulmonary function tests[114]. This would provide for faster and more widespread screening and early diagnosis of COVID.
(11) It is necessary to develop standardized protocols for reporting and sharing disease-related multi-source data across healthcare facilities. Standardizing electronic health records (EHRs) and telemedicine platforms would allow for them to be leveraged to facilitate swift and secure information exchange while ensuring privacy protection and data security.
(12) It is important to provide a secure healthcare and data sharing environment when taking management strategies such as digital passports. Ethical considerations and regulatory and privacy-preservation constraints must be considered. Currently, sovereign liability laws are not at all AI-ready. Therefore, the liability system needs to be adjusted or patients, customers, and AI developers will all be harmed by poor liability policies.
In summary, wearables offer tremendous potential to provide quick reactions to outbreaks and better care for the public by combining mHealth and AI technology. However, there are still limitations and challenges that need to be addressed for clinical-grade reliability for successful implementation in pandemic management scenarios. Primary concerns revolve around data privacy, data security, and data ownership, as wearable devices collect sensitive physiological information that needs to be handled with utmost care to prevent unauthorized access or breaches[12]. Balancing individual rights to privacy with public health will be particularly important during specific situations. The reliability of AI algorithms in interpreting data accurately and providing meaningful insights is contingent upon the quality and diversity of the training data available. It is important to note that the clinical design and implementation of these wearables were conducted in resource-rich settings, which can, in turn, create intrinsic biases in the dataset[132]. Moreover, bias in the training data can lead to skewed predictions and hinder the algorithm’s generalizability. Regarding hardware development, the use of healthcare wearables remains in relatively early stages of development. Further improvements are still required in sensor performance, accuracy, user comfort, and other related aspects. Additionally, factors such as user compliance, device calibration, and potential technical glitches could affect the reliability and accuracy of the collected data. These challenges highlight the necessity for adopting a holistic approach that encompasses regulatory considerations, technological advancements, and user-oriented design principles for effective implementation. If the suggested system and associated technologies could be fully constructed and distributed, it would be possible to achieve prediction and early detection of COVID, improve clinical diagnosis, and provide patient-centered long COVID care. Additionally, these approaches would offer prompt medical assistance, teleconsultation, telemedicine, user-oriented healthcare, etc., thereby improving pandemic response capabilities and lightening the burden on healthcare professionals and institutions. Multidisciplinary collaboration between scientists, healthcare professionals, engineers, and policymakers is essential to design, verify, and upgrade the system for handling future pandemics. The proposed system could broaden utilizations in various diseases beyond COVID-19, possessing the capacity to revolutionize the existing healthcare framework and generate far-reaching implications, elevating it to unprecedented levels.
DECLARATIONS
Acknowledgments
We acknowledge the responses generated by ChatGPT on the questions regarding early diagnosis, control, and management of COVID-19 and possible future pandemics. These insights support and align closely with the proposed responding system we intend to introduce. Additionally, we also acknowledge its contributions to improving the readability and language of our manuscript. D. A. Clifton was supported by the Pandemic Sciences Institute at the University of Oxford; the National Institute for Health Research (NIHR) Oxford Biomedical Research Centre (BRC); an NIHR Research Professorship; a Royal Academy of Engineering Research Chair; and the InnoHK Hong Kong Centre for Centre for Cerebro-cardiovascular Engineering (COCHE).
Authors’ contributions
Contributed to the literature review, responding system design and conception: Xiang T, Liu Z, Ji N, Zhang YT
Involved in the manuscript preparation and writing: Xiang T, Liu Z, Ji N, Veetil JC, Zhu H
Provided insights and professional guidance and improvement on the application of AI on the responding system and the manuscript: Lu L, Clifton DA, Deen MJ, Lovell NH
Provided clinical insights and guidance on the potential applications of wearables on the responding system: Yan B, Mok V, Li X
Made substantial contributions to the conception of this topic and provided overall guidance on the manuscript writing: Zhang YT
Availability of data and materials
Not applicable.
Financial support and sponsorship
This work was supported by the ITC-InnoHK grant to Hong Kong Centre for Cerebro-cardiovascular Health Engineering (COCHE) and City University of Hong Kong (CityU) starting up grant.
Conflicts of interest
All authors declared that there are no conflicts of interest.
Ethical approval and consent to participate
Not applicable.
Consent for publication
Not applicable.
Copyright
© The Author(s) 2023.
APPENDIX
ChatGPT full responses to the questions regarding early diagnosis, control and management of COVID-19 and possible future pandemics
Question | ChatGPT response |
What specific measures or actions can you effectively undertake when facing COVID-19? | As an AI language model, I don't have personal experiences or physical capabilities, so I cannot directly face or interact with COVID-19. However, I can provide information, answer questions, and assist with various aspects related to the COVID-19 pandemic. Here are some ways I can be helpful: 1. Provide information: I can offer up-to-date information about COVID-19, including symptoms, prevention measures, and current guidelines from health organizations 2. Answer questions: You can ask me any specific questions you have about COVID-19, such as its transmission, testing methods, available treatments, or vaccine development 3. Clarify misconceptions: I can help debunk common myths or misinformation surrounding COVID-19 and provide accurate information based on scientific research and reliable sources 4. Offer guidance: If you're seeking advice on how to stay safe and protect yourself and others, I can provide general recommendations, such as practicing good hygiene, wearing masks, and following social distancing guidelines 5. Provide updates: I can offer updates on the global COVID-19 situation, including statistics, new variants, vaccination progress, and any significant developments in research or public health measures 6. Emotional support: Dealing with a pandemic can be stressful. I can provide emotional support by offering reassurance, coping strategies, and mental health resources to help you navigate the challenges of the situation Remember, while I strive to provide accurate and helpful information, it's always important to consult official sources and healthcare professionals for specific medical advice or urgent concerns regarding COVID-19 |
What specific information would you require to enable early diagnosis of COVID-19 and provide effective solutions for controlling and managing the spread of the virus in its earliest phase? | To enable early diagnosis of COVID-19 and provide solutions to control and manage its spread in the earliest phase, the following information would be helpful: 1. Symptoms: Detailed information about common and atypical symptoms associated with COVID-19, such as fever, cough, shortness of breath, fatigue, loss of taste or smell, muscle aches, sore throat, headache, gastrointestinal symptoms., etc 2. Risk factors: Factors that may increase the risk of COVID-19, including age, underlying health conditions (such as diabetes, heart disease, respiratory issues), immunocompromised status, occupational exposure, recent travel history, or close contact with confirmed cases 3. Diagnostic tests: Information on available diagnostic tests for COVID-19, such as PCR (Polymerase Chain Reaction) tests, rapid antigen tests, antibody tests, or any emerging diagnostic technologies. This includes details about their accuracy, turnaround time, availability, and recommended testing protocols 4. Testing strategies: Guidelines or recommendations regarding testing strategies, such as targeted testing based on symptoms, contact tracing, surveillance testing in high-risk settings or populations, and the integration of health data from wearables for early detection 5. Public health measures: Information on effective public health measures to control and manage the spread of COVID-19, including guidelines on mask usage, hand hygiene, social distancing, ventilation, travel restrictions, quarantine protocols, and vaccination strategies. Integration of health data from wearables can provide additional insights for personalized preventive measures 6. Contact tracing: Strategies and technologies for effective contact tracing, including digital contact tracing apps, manual contact tracing protocols, or a combination of both. Incorporating health data from wearables can enhance contact tracing efforts by providing information on proximity to infected individuals 7. Vaccination: Updates on COVID-19 vaccination efforts, including information on vaccine distribution, prioritization strategies, vaccine efficacy, potential side effects, booster shots, and vaccination coverage goals. Wearable health data can assist in monitoring vaccination status and effectiveness 8. Treatment options: Information on approved treatments for COVID-19, including antiviral medications, monoclonal antibodies, and supportive care measures. Updates on emerging treatments or therapies under investigation, along with the integration of wearable health data, can contribute to personalized treatment approaches 9. Surveillance and monitoring systems: Information on surveillance systems and tools used to monitor the spread of COVID-19, track cases, and identify hotspots. Incorporating health data from wearables into surveillance systems can enhance real-time monitoring and early detection 10. Public communication strategies: Guidelines for effective public communication and risk messaging, including strategies to combat misinformation, promote adherence to preventive measures, and address vaccine hesitancy. Leveraging health data from wearables can aid in targeted communication efforts based on individual risk profiles By integrating health data from wearables with comprehensive and up-to-date information on these aspects, it would be possible to improve early diagnosis, implement appropriate control measures, and effectively manage the spread of COVID-19 in its earliest phase while considering personalized health insights |
What kind of system would you suggest for the early detection of future pandemics? | I would suggest implementing a comprehensive system for the early detection of future pandemics. This system should involve the following components: 1. Global Surveillance Network: Establish a global network that monitors potential disease outbreaks by fostering collaboration between countries, international health organizations, and local healthcare systems. Real-time data and information sharing would be essential 2. Advanced Data Analysis: Develop advanced algorithms and artificial intelligence (AI) systems capable of analyzing diverse data sources, such as social media, news reports, hospital records, and animal health data. These systems should be designed to detect early signs of potential outbreaks or unusual disease patterns 3. Integrated Healthcare Systems: Integrate healthcare systems, including hospitals, clinics, and laboratories, to facilitate seamless data collection and analysis. Standardized protocols for reporting and sharing disease-related data should be implemented, utilizing electronic health records (EHRs) and telemedicine platforms 4. Animal Surveillance: Strengthen surveillance of animal populations, particularly those known to harbor potential zoonotic diseases. Enhanced monitoring of wildlife, livestock, and domesticated animals can help identify potential spillover events and prevent the transmission of diseases to humans 5. Enhanced Biosurveillance at Borders: Strengthen screening procedures at international borders, including airports, seaports, and land crossings. This involves implementing advanced screening technologies, such as thermal scanners, rapid diagnostic tests, and AI-based risk assessment tools, to identify potential cases and high-risk travelers 6. Public Health Education: Invest in public health education programs to raise awareness and improve reporting of potential disease outbreaks Encouraging individuals to seek medical attention for unusual symptoms or patterns of illness, promoting hygiene practices, and implementing early vaccination campaigns are important preventive measures 7. Global Cooperation and Funding: Foster international cooperation and secure sustainable funding to support early detection efforts Collaboration with governments, multilateral organizations, philanthropic foundations, and the private sector is crucial in establishing dedicated funds for pandemic preparedness and response. 8. Research and Development: Invest in research and development for innovative diagnostic tools, vaccines, antiviral therapies, and other medical interventions. Prioritizing research on emerging infectious diseases, involving academic institutions, pharmaceutical companies, and biotechnology firms, is essential 9. Multidisciplinary Approach: Encourage collaboration among experts from diverse fields such as epidemiology, virology, public health, data science, and social sciences. Interdisciplinary research and knowledge exchange can provide a comprehensive understanding of disease dynamics and risk factors By implementing such a system, with a focus on global collaboration, information sharing, advanced analytics, and early intervention, we can significantly improve our ability to detect and respond to future pandemics at their earliest stages. |
What roles can physiological and molecular sensing play in the prediction and early detection of future pandemics? | Physiological and molecular sensing can play crucial roles in the prediction and early detection of future pandemics. Here are some ways these sensing technologies can contribute: 1. Disease Biomarker Identification: Physiological and molecular sensing techniques can help identify specific biomarkers associated with infectious diseases. By analyzing bodily fluids, such as blood, saliva, or urine, these sensors can detect the presence of pathogens or changes in biomarker levels that indicate the onset of a disease. This information can aid in early detection and prediction of a potential pandemic 2. Rapid Diagnostic Tools: Portable and rapid diagnostic devices equipped with physiological and molecular sensing capabilities can be deployed in various settings, including clinics, community centers, and even homes. These devices can detect specific viral or bacterial genetic material, proteins, or antibodies, providing quick and accurate results. Point-of-care diagnostics enable early identification of infected individuals and facilitate prompt public health interventions 3. Point-of-Care Diagnostics: Portable and rapid diagnostic devices equipped with physiological and molecular sensing capabilities can be deployed in various settings, including clinics, community centers, and even homes. These devices can detect specific viral or bacterial genetic material, proteins, or antibodies, providing quick and accurate results. Point-of-care diagnostics enable early identification of infected individuals and facilitate prompt public health interventions 4. Wearable Devices: Physiological sensing through wearable devices, such as smartwatches or fitness trackers, can provide continuous monitoring of vital signs and health parameters. These devices can track changes in body temperature, heart rate, respiratory rate, and oxygen saturation levels, which can serve as early indicators of an infectious disease outbreak. Real-time data from wearables can be collected and analyzed to identify unusual patterns or deviations from the norm, triggering alerts for further investigation 5. Environmental Monitoring: Molecular sensing technologies can be used to monitor environmental factors that contribute to disease transmission. For example, sensors can detect the presence of specific pathogens or toxins in air, water, or soil samples. By continuously monitoring high-risk areas or regions with known disease reservoirs, early warnings can be issued, enabling timely intervention and prevention measures 6. Syndromic Surveillance: By integrating physiological and molecular sensing technologies with existing surveillance systems, comprehensive syndromic surveillance can be achieved. Real-time data from wearable devices, point-of-care diagnostics, and other sensing platforms can be aggregated and analyzed alongside traditional epidemiological data sources. This integration enables the detection of unusual disease patterns or clusters of symptoms, providing early warning signals for potential pandemics 7. Remote Monitoring and Telemedicine: Physiological sensing technologies enable remote monitoring of individuals who may be at risk or already infected. Telemedicine platforms can integrate sensors to monitor symptoms, vital signs, and disease progression in real time. This approach allows healthcare providers to remotely assess patients, provide timely guidance, and initiate appropriate interventions, minimizing the risk of disease transmission and easing the burden on healthcare systems 8. Early Warning Systems: Physiological and molecular sensing data can be combined with advanced analytics and machine learning algorithms to develop early warning systems for pandemics. By continuously monitoring large-scale data streams from multiple sources, such systems can identify anomalies, detect emerging patterns, and predict the likelihood of a disease outbreak before it becomes widespread Integration of physiological and molecular sensing technologies into the early detection and prediction of future pandemics enhances our ability to identify and respond to infectious diseases promptly. These sensing approaches provide valuable data for surveillance, diagnostics, and public health interventions, ultimately helping to mitigate the impact of pandemics on global health |
REFERENCES
1. WHO Coronavirus (COVID-19) Dashboard. Available from: https://covid19.who.int [Last accessed on 25 Aug 2023].
2. WHO chief says China's zero-Covid strategy is 'unsustainable'. Available from: https://www.france24.com/en/asia-pacific/20220511-who-chief-says-china-s-zero-covid-strategy-is-unsustainable [Last accessed on 25 Aug 2023].
3. Rai PK, Mueed Z, Chowdhury A, et al. Current overviews on COVID-19 management strategies. Curr Pharm Biotechnol 2022;23:361-87.
4. COVID-19 resurgence hits but China not to return to lockdown approach: report. Available from: https://www.msn.com/en-in/health/health-news/covid-19-resurgence-hits-but-china-not-to-return-to-lockdown-approach-report/ar-AA1bP4E4 [Last accessed on 29 Aug 2023].
5. Possas C, Marques ETA, Risi JB Jr, Homma A. COVID-19 and future Disease X in circular economy transition: redesigning pandemic preparedness to prevent a global disaster. Circ Econ Sustain 2021;1:1463-78.
6. Ye Q, Zhou J, Wu H. Using information technology to manage the COVID-19 pandemic: development of a technical framework based on practical experience in China. JMIR Med Inform 2020;8:e19515.
7. Thoradeniya T, Jayasinghe S. COVID-19 and future pandemics: a global systems approach and relevance to SDGs. Global Health 2021;17:59.
8. Bayoumy K, Gaber M, Elshafeey A, et al. Smart wearable devices in cardiovascular care: where we are and how to move forward. Nat Rev Cardiol 2021;18:581-99.
9. Cheong SHR, Ng YJX, Lau Y, Lau ST. Wearable technology for early detection of COVID-19: a systematic scoping review. Prev Med 2022;162:107170.
10. Channa A, Popescu N, Skibinska J, Burget R. The rise of wearable devices during the covid-19 pandemic: a systematic review. Sensors 2021;21:5787.
11. Khondakar KR, Kaushik A. Role of wearable sensing technology to manage long COVID. Biosensors 2022;13:62.
12. Ding X, Clifton D, Ji N, et al. Wearable sensing and telehealth technology with potential applications in the coronavirus pandemic. IEEE Rev Biomed Eng 2021;14:48-70.
13. Adans-Dester CP, Bamberg S, Bertacchi FP, et al. Can mHealth technology help mitigate the effects of the COVID-19 pandemic? IEEE Open J Eng Med Biol 2020;1:243-8.
14. Symptoms of COVID-19. Available from: https://www.cdc.gov/coronavirus/2019-ncov/symptoms-testing/symptoms.html [Last accessed on 25 Aug 2023].
15. Is fatigue a symptom of COVID-19? Available from: https://health-study.joinzoe.com/post/covid-symptoms-fatigue-tiredness [Last accessed on 25 Aug 2023].
16. Coronavirus symptoms: pain in THESE two areas could be an early sign of COVID. Available from: https://timesofindia.indiatimes.com/life-style/health-fitness/health-news/coronavirus-symptoms-pain-in-these-two-areas-could-be-an-early-sign-of-covid/photostory/92487056.cms [Last accessed on 25 Aug 2023].
17. Cardiac symptoms can be first sign of COVID-19. Available from: https://www.mdedge.com/cardiology/article/219645/coronavirus-updates/cardiac-symptoms-can-be-first-sign-covid-19 [Last accessed on 25 Aug 2023].
18. Post COVID-19 condition (Long COVID). Available from: https://www.who.int/europe/news-room/fact-sheets/item/post-covid-19-condition [Last accessed on 25 Aug 2023].
19. UCSF research highlights Oura’s potential to identify fevers and aid in early illness detection of COVID-19 and other illnesses. Available from: https://ouraring.com/blog/tempredict_covid19_research/ [Last accessed on 25 Aug 2023].
20. Track your nightly wrist temperature changes with Apple Watch. Available from: https://support.apple.com/en-hk/HT213275 [Last accessed on 25 Aug 2023].
21. Meet the world’s first smart wrist band that can detect body temperature. Available from: https://indianexpress.com/article/technology/tech-news-technology/meet-the-worlds-first-smart-wrist-band-that-can-detect-body-temperature/ [Last accessed on 25 Aug 2023].
22. Un KC, Wong CK, Lau YM, et al. Observational study on wearable biosensors and machine learning-based remote monitoring of COVID-19 patients. Sci Rep 2021;11:4388.
23. Suo J, Liu Y, Wu C, et al. Wide-bandwidth nanocomposite-sensor integrated smart mask for tracking multiphase respiratory activities. Adv Sci 2022;9:e2203565.
24. Jeong H, Lee JY, Lee K, et al. Differential cardiopulmonary monitoring system for artifact-canceled physiological tracking of athletes, workers, and COVID-19 patients. Sci Adv 2021;7:eabg3092.
25. Emokpae LE, Emokpae RN, Lalouani W, Younis M. Smart multimodal telehealth-iot system for COVID-19 patients. IEEE Pervasive Comput 2021;20:73-80.
26. Pal A, Sankarasubbu M. Pay attention to the cough: early diagnosis of COVID-19 using interpretable symptoms embeddings with cough sound signal processing. Proceedings of the 36th Annual ACM Symposium on Applied Computing(SAC '21). Association for Computing Machinery, New York, NY, USA; 2021.p. 620-8
27. Nachiar CC, Ambika N, MoulikaR, Poovendran R. Design of cost-effective wearable sensors with integrated health monitoring system. 2020 Fourth International Conference on I-SMAC (IoT in Social, Mobile, Analytics and Cloud)(I-SMAC). Palladam, India; 2020.p. 1289-92.
28. Zhong J, Li Z, Takakuwa M, et al. Smart face mask based on an ultrathin pressure sensor for wireless monitoring of breath conditions. Adv Mater 2022;34:e2107758.
29. Ji Z, Zhang M. Highly sensitive and stretchable piezoelectric strain sensor enabled wearable devices for real-time monitoring of respiratory and heartbeat simultaneously. Nanotechnol Precis Eng 2022;5:013002.
30. Li Y, Liu C, Zou H, et al. Integrated wearable smart sensor system for real-time multi-parameter respiration health monitoring. Cell Rep Phys Sci 2023;4:101191.
31. Leveraging WHOOP technology to predict COVID-19 risk. Available from: https://www.whoop.com/eu/en/thelocker/predict-covid-19-risk/ [Last accessed on 29 Aug 2023].
32. Xu S, Kim J, Walter JR, Ghaffari R, Rogers JA. Translational gaps and opportunities for medical wearables in digital health. Sci Transl Med 2022;14:eabn6036.
33. Klum M, Urban M, Tigges T, et al. Wearable cardiorespiratory monitoring employing a multimodal digital patch stethoscope: estimation of ECG, PEP, LVET and respiration using a 55 mm single-lead ECG and phonocardiogram. Sensors 2020;20:2033.
34. Mason AE, Hecht FM, Davis SK, et al. Detection of COVID-19 using multimodal data from a wearable device: results from the first TemPredict study. Sci Rep 2022;12:3463.
35. Emokpae LE, Emokpae RN Jr, Bowry E, et al. A wearable multi-modal acoustic system for breathing analysis. J Acoust Soc Am 2022;151:1033.
36. 10 best smartwatches and fitness trackers with oxygen monitors. Available from: https://www.online-tech-tips.com/gadgets/10-best-smartwatches-and-fitness-trackers-with-oxygen-monitors/ [Last accessed on 25 Aug 2023].
37. Astroskin space-grade smart garments. Available from: https://www.hexoskin.com/pages/astroskin-vital-signs-monitoring-platform-for-advanced-research [Last accessed on 25 Aug 2023].
38. Yabuki R, Qian Z, Lee KM, et al. PPG and SpO2 recording circuit with ambient light cancellation for trans-nail pulse-wave monitoring system. 2019 IEEE Biomedical Circuits and Systems Conference (BioCAS). Nara, Japan; 2019.p. 1-4
39. Davies HJ, Williams I, Peters NS, Mandic DP. In-Ear SpO2: a tool for wearable, unobtrusive monitoring of core blood oxygen saturation. Sensors 2020;20:4879.
40. Huang X, Li X, Wu Z, et al. Techniques and materials for highthroughput-fabrication and integration of multiparameter flexible skin patches. Adv Mater Technol-Us 2023;8:2201051.
41. Takeshita T, Yoshida M, Takei Y, et al. Development of wearable multi-lead ECG measurement device using cubic flocked electrode. Sci Rep 2022;12:19308.
42. Yang J, Zhang K, Yu J, et al. Facile fabrication of robust and reusable PDMS supported graphene dry electrodes for wearable electrocardiogram monitoring. Adv Mater Technol 2021;6:2100262.
43. Zheng Y, Li Y, Zhao Y, et al. Ultrathin and highly breathable electronic tattoo for sensing multiple signals imperceptibly on the skin. Nano Energy 2023;107:108092.
44. Ishii K, Hiraoka N. Nail tip sensor: toward reliable daylong monitoring of heart rate. IEEJ T T Electr Electr 2020;15:902-8.
45. Guo B, Tang H, Xia S, Wang M, Hu Y, Zhao Z. Development of a multi-channel wearable heart sound visualization system. J Pers Med 2022;12:2011.
46. Ximing H, Notsu S, Choi D, Siriaraya P, Kuwahara N. Development of wearable heart sound collection device. Int J Adv Comput Sc 2021;12:online ahead of print.
47. Luo H, Lamata P, Bazin S, et al. Smartphone as an electronic stethoscope: factors influencing heart sound quality. Eur Heart J Digit Health 2022;3:473-80.
48. Biobeat Home. Available from: https://www.bio-beat.com/ [Last accessed on 25 Aug 2023].
49. Vital. Verified. Valencell. Available from: https://valencell.com/ [Last accessed on 25 Aug 2023].
50. Lee KM, Qian Z, Yabuki R, et al. Continuous peripheral blood pressure measurement with ECG and PPG signals at fingertips. 2018 IEEE Biomedical Circuits and Systems Conference (BioCAS). Cleveland, OH, USA; 2018.p. 1-4.
51. Wang S, Xiao J, Liu H, Zhang L. Silk nanofibrous iontronic sensors for accurate blood pressure monitoring. Chem Eng J 2023;453:139815.
52. Kireev D, Sel K, Ibrahim B, et al. Continuous cuffless monitoring of arterial blood pressure via graphene bioimpedance tattoos. Nat Nanotechnol 2022;17:864-70.
53. Truong H, Montanari A, Kawsar F. Non-invasive blood pressure monitoring with multi-modal in-ear sensing. ICASSP 2022-2022 IEEE International Conference on Acoustics, Speech and Signal Processing (ICASSP). Singapore; 2022.p. 6-10.
54. Jeong H, Yoo JY, Ouyang W, et al. Closed-loop network of skin-interfaced wireless devices for quantifying vocal fatigue and providing user feedback. Proc Natl Acad Sci USA 2023;120:e2219394120.
55. Schweizer T, Wyss T, Gilgen-Ammann R. Detecting soldiers' fatigue using eye-tracking glasses: practical field applications and research opportunities. Mil Med 2022;187:e1330-7.
56. Honiball JR, Vandenheever D. The development of a PPG and in-ear EEG device for application in fatigue measurement. Am J Sci Eng 2022;3:7-17.
57. Mapelli M, Vignati C, Gugliandolo P, Fumagalli D, Agostoni P. Feasibility of remote home monitoring with a T-shirt wearable device in post-recovery COVID-19 patients. J Cardiovasc Med 2021;22:860-3.
58. Cheung J, Leary EB, Lu H, Zeitzer JM, Mignot E. PSG validation of minute-to-minute scoring for sleep and wake periods in a consumer wearable device. PLoS One 2020;15:e0238464.
59. Pham N, Dinh T, Raghebi Z, et al. WAKE: a behind-the-ear wearable system for microsleep detection. Proceedings of the 18th International Conference on Mobile Systems, Applications, and Services (MobiSys '20). New York, NY, USA; 2020.p. 404-18.
60. Troubleshooting the mystery of post-COVID insomnia with a sleep tracker. Available from: https://mashable.com/article/sleep-tracker-insomnia-covid-side-effect [Last accessed on 25 Aug 2023].
61. Coronavirus resource center. Available from: https://www.health.harvard.edu/diseases-and-conditions/if-youve-been-exposed-to-the-coronavirus#:~:text=A%20person%20with%20COVID%2D19,behaviors%20designed%20to%20prevent%20spread [Last accessed on 25 Aug 2023].
62. Coronavirus disease (COVID-19): how is it transmitted? Available from: https://www.who.int/news-room/questions-and-answers/item/coronavirus-disease-covid-19-how-is-it-transmitted [Last accessed on 25 Aug 2023].
63. Early symptoms of COVID-19: what you need to know. Available from: https://www.medicalnewstoday.com/articles/coronavirus-early-symptoms [Last accessed on 25 Aug 2023].
64. Smarr BL, Aschbacher K, Fisher SM, et al. Feasibility of continuous fever monitoring using wearable devices. Sci Rep 2020;10:21640.
65. Miller DJ, Capodilupo JV, Lastella M, et al. Analyzing changes in respiratory rate to predict the risk of COVID-19 infection. PLoS One 2020;15:e0243693.
66. Chen A, Rhoades RD, Halton AJ, et al. Wireless wearable ultrasound sensor to characterize respiratory behavior. In: Ossandon MR, Baker H, Rasooly A, editors. Biomedical engineering technologies. New York: Springer US; 2022. pp. 671-82.
67. Liu Y, Zhao L, Avila R, et al. Epidermal electronics for respiration monitoring via thermo-sensitive measuring. Mater Today Phys 2020;13:100199.
68. Greenhalgh T, Knight M, Inda-Kim M, Fulop NJ, Leach J, Vindrola-Padros C. Remote management of covid-19 using home pulse oximetry and virtual ward support. BMJ 2021;372:n677.
69. Clerkin KJ, Fried JA, Raikhelkar J, et al. COVID-19 and cardiovascular disease. Circulation 2020;141:1648-55.
70. Kaliyaperumal D, Bhargavi K, Ramaraju K, Nair KS, Ramalingam S, Alagesan M. Electrocardiographic changes in COVID-19 patients: a hospital-based descriptive study. Indian J Crit Care Med 2022;26:43-8.
71. Naghshvarianjahromi M, Majumder S, Kumar S, Naghshvarianjahromi N, Deen MJ. Natural brain-inspired intelligence for screening in healthcare applications. IEEE Access 2021;9:67957-73.
72. Majumder S, Chen L, Marinov O, Chen CH, Mondal T, Deen MJ. Noncontact wearable wireless ECG systems for long-term monitoring. IEEE Rev Biomed Eng 2018;11:306-21.
73. Nemati E, Deen M, Mondal T. A wireless wearable ECG sensor for long-term applications. IEEE Commun Mag 2012;50:36-43.
74. British Heart Foundation. How does Covid-19 affect your heart? Available from: https://www.bhf.org.uk/informationsupport/heart-matters-magazine/news/coronavirus-and-your-health/what-does-coronavirus-do-to-your-body [Last accessed on 25 Aug 2023].
75. Shandhi MMH, Cho PJ, Roghanizad AR, et al. A method for intelligent allocation of diagnostic testing by leveraging data from commercial wearable devices: a case study on COVID-19. NPJ Digit Med 2022;5:130.
76. Poongodi M, Hamdi M, Malviya M, Sharma A, Dhiman G, Vimal S. Retracted article: diagnosis and combating COVID-19 using wearable Oura smart ring with deep learning methods. Pers Ubiquitous Comput 2022;26:25-35.
77. Sel K, Osman D, Huerta N, Edgar A, Pettigrew RI, Jafari R. Continuous cuffless blood pressure monitoring with a wearable ring bioimpedance device. NPJ Digit Med 2023;6:59.
78. Gnanvi JE, Salako KV, Kotanmi GB, Glèlè Kakaï R. On the reliability of predictions on Covid-19 dynamics: a systematic and critical review of modelling techniques. Infect Dis Model 2021;6:258-72.
79. Khodeir MM, Shabana HA, Alkhamiss AS, et al. Early prediction keys for COVID-19 cases progression: a meta-analysis. J Infect Public Health 2021;14:561-9.
80. Mishra T, Wang M, Metwally AA, et al. Pre-symptomatic detection of COVID-19 from smartwatch data. Nat Biomed Eng 2020;4:1208-20.
81. Empatica receives first of its kind European CE mark for early detection of COVID-19. Available from: https://www.empatica.com/blog/aura-and-care-receive-ce-mark-for-early-detection-of-covid-19-and-other-respiratory-infections.html [Last accessed on 25 Aug 2023].
82. Coronavirus (COVID-19) update: FDA authorizes first machine learning-based screening device to identify certain biomarkers that may indicate COVID-19 infection. Available from: https://www.fda.gov/news-events/press-announcements/coronavirus-covid-19-update-fda-authorizes-first-machine-learning-based-screening-device-identify [Last accessed on 25 Aug 2023].
83. COVID plus prescreening monitor. Available from: https://www.covidplusmonitor.com/ [Last accessed on 25 Aug 2023].
84. Nguyen PQ, Soenksen LR, Donghia NM, et al. Wearable biosensors enabled by cell-free synthetic biology. Available from: https://hdl.handle.net/1721.1/131278] [Last accessed on 25 Aug 2023].
85. Nguyen PQ, Soenksen LR, Donghia NM, et al. Wearable materials with embedded synthetic biology sensors for biomolecule detection. Nat Biotechnol 2021;39:1366-74.
86. Yunlong Cao COVID predictor. Available from: https://pesquisa.bvsalud.org/global-literature-on-novel-coronavirus-2019-ncov/resource/pt/covidwho-2185685] [Last accessed on 25 Aug 2023].
87. Tadic M, Saeed S, Grassi G, Taddei S, Mancia G, Cuspidi C. Hypertension and COVID-19: ongoing controversies. Front Cardiovasc Med 2021;8:639222.
88. Laffin LJ, Kaufman HW, Chen Z, et al. Rise in blood pressure observed among US adults during the COVID-19 pandemic. Circulation 2022;145:235-7.
89. Liu J, Qiu S, Luo N, et al. PCA-based multi-wavelength photoplethysmography algorithm for cuffless blood pressure measurement on elderly subjects. IEEE J Biomed Health Inform 2021;25:663-73.
90. Indraratna P, Biswas U, Yu J, et al. Trials and tribulations: mhealth clinical trials in the COVID-19 pandemic. Yearb Med Inform 2021;30:272-9.
92. What to know about long COVID. Available from: https://www.medicalnewstoday.com/articles/long-covid#symptoms [Last accessed on 25 Aug 2023].
94. Khan S, Siddique R, Li H, et al. Impact of coronavirus outbreak on psychological health. J Glob Health 2020;10:010331.
95. Targa ADS, Benítez ID, Moncusí-Moix A, et al. Decrease in sleep quality during COVID-19 outbreak. Sleep Breath 2021;25:1055-61.
96. Spierer DK, Rosen Z, Litman LL, Fujii K. Validation of photoplethysmography as a method to detect heart rate during rest and exercise. J Med Eng Technol 2015;39:264-71.
97. Fonseca P, Long X, Radha M, Haakma R, Aarts RM, Rolink J. Sleep stage classification with ECG and respiratory effort. Physiol Meas 2015;36:2027-40.
98. Willemen T, Van Deun D, Verhaert V, et al. An evaluation of cardiorespiratory and movement features with respect to sleep-stage classification. IEEE J Biomed Health Inform 2014;18:661-9.
99. Mekhael M, Lim CH, El Hajjar AH, et al. Studying the effect of long COVID-19 infection on sleep quality using wearable health devices: observational study. J Med Internet Res 2022;24:e38000.
102. DePace NL, Colombo J. Long-COVID syndrome and the cardiovascular system: a review of neurocardiologic effects on multiple systems. Curr Cardiol Rep 2022;24:1711-26.
103. Davenport T, Kalakota R. The potential for artificial intelligence in healthcare. Fut Healthc J 2019;6:94-8.
104. Almalki M, Azeez F. Health chatbots for fighting COVID-19: a scoping review. Acta Inform Med 2020;28:241-7.
105. Make critical decisions with clarity and confidence. Available from: https://bluedot.global [Last accessed on 25 Aug 2023].
106. The disease daily. Available from: https://www.diseasedaily.org/about/ [Last accessed on 25 Aug 2023].
107. Satpathy S, Nandan Mohanty S, Chatterjee JM, Swain A. Comprehensive claims of AI for healthcare applications-coherence towards COVID-19. Appl Artif Intell COVID-19 2021:3-18.
108. Xue VW, Lei P, Cho WC. The potential impact of ChatGPT in clinical and translational medicine. Clin Transl Med 2023;13:e1216.
109. Sedaghat S. Early applications of ChatGPT in medical practice, education and research. Clin Med 2023;23:278-9.
110. Khan RA, Jawaid M, Khan AR, Sajjad M. ChatGPT-reshaping medical education and clinical management. Pak J Med Sci 2023;39:605-7.
111. New and surprising evidence that ChatGPT can perform several intricate tasks relevant to handling complex medical and clinical information. Available from: https://www.news-medical.net/news/20230213/New-and-surprising-evidence-that-ChatGPT-can-perform-several-intricate-tasks-relevant-to-handling-complex-medical-and-clinical-information.aspx [Last accessed on 25 Aug 2023].
112. ChatGPT passes US medical licensing exam without clinician input. Available from: https://healthitanalytics.com/news/chatgpt-passes-us-medical-licensing-exam-without-clinician-input [Last accessed on 25 Aug 2023].
113. Sng GGR, Tung JYM, Lim DYZ, Bee YM. Potential and pitfalls of ChatGPT and natural-language artificial intelligence models for diabetes education. Diabetes Care 2023;46:e103-5.
114. Jiang W, Majumder S, Kumar S, et al. A wearable tele-health system towards monitoring covid-19 and chronic diseases. IEEE Rev Biomed Eng 2022;15:61-84.
115. Jorfi M, Luo NM, Hazra A, et al. Diagnostic technology for COVID-19: comparative evaluation of antigen and serology-based SARS-CoV-2 immunoassays, and contact tracing solutions for potential use as at-home products. MedRxiv 2020:online ahead of print.
116. Ferretti L, Wymant C, Kendall M, et al. Quantifying SARS-CoV-2 transmission suggests epidemic control with digital contact tracing. Science 2020;368:eabb6936.
117. Amft O, Lopera L, Lukowicz P, Bian S, Burggraf P. Wearables to fight COVID-19: from symptom tracking to contact tracing. IEEE Pervasive Comput 2020;19:53-60.
118. Ye F, Majumder S, Jiang W, et al. A framework for infectious disease monitoring with automated contact tracing-a case study of COVID-19. IEEE Internet Things J 2023;10:144-65.
119. Dar AB, Lone AH, Zahoor S, Khan AA, Naaz R. Applicability of mobile contact tracing in fighting pandemic (COVID-19): issues, challenges and solutions. Comput Sci Rev 2020;38:100307.
120. Santos MD, Roman C, Pimentel MAF, et al. A real-time wearable system for monitoring vital signs of COVID-19 patients in a hospital setting. Front Digit Health 2021;3:630273.
121. National Wastewater Surveillance System (NWSS). Available from: https://www.cdc.gov/nwss/wastewater-surveillance.html [Last accessed on 25 Aug 2023].
122. Deng Y, Xu X, Zheng X, et al. Use of sewage surveillance for COVID-19 to guide public health response: a case study in Hong Kong. Sci Total Environ 2022;821:153250.
123. Polo D, Quintela-Baluja M, Corbishley A, et al. Making waves: wastewater-based epidemiology for COVID-19-approaches and challenges for surveillance and prediction. Water Res 2020;186:116404.
124. Lahrich S, Laghrib F, Farahi A, Bakasse M, Saqrane S, El Mhammedi MA. Review on the contamination of wastewater by COVID-19 virus: impact and treatment. Sci Total Environ 2021;751:142325.
125. Ji N, Xiang T, Bonato P, et al. Recommendation to use wearable-based mhealth in closed-loop management of acute cardiovascular disease patients during the COVID-19 pandemic. IEEE J Biomed Health Inform 2021;25:903-8.
126. A strong tool for the reopening of our economies and societies. Available from: https://commission.europa.eu/strategy-and-policy/coronavirus-response/safe-covid-19-vaccines-europeans/eu-digital-covid-certificate_en [Last accessed on 25 Aug 2023].
127. The European Commission and WHO launch landmark digital health initiative to strengthen global health security. Available from: https://www.who.int/news/item/05-06-2023-the-european-commission-and-who-launch-landmark-digital-health-initiative-to-strengthen-global-health-security [Last accessed on 25 Aug 2023].
128. Jackson EB, Dreyling, Pappel I. Challenges and Implications of the WHO’s Digital Cross-Border COVID-19 Vaccine Passport Recognition Pilot. 2021 Eighth International Conference on eDemocracy & eGovernment (ICEDEG). Quito, Ecuador; 2021.p. 28-30.
129. Mbunge E, Fashoto S, Batani J. COVID-19 digital vaccination certificates and digital technologies: lessons from digital contact tracing apps. Available from: https://www.researchgate.net/profile/John-Batani/publication/350200900_COVID-19_Digital_Vaccination_Certificates_and_Digital_Technologies_Lessons_from_Digital_Contact_Tracing_Apps/links/61a1f7686b9a6f096708b6b1/COVID-19-Digital-Vaccination-Certificates-and-Digital-Technologies-Lessons-from-Digital-Contact-Tracing-Apps.pdf [Last accessed on 25 Aug 2023].
130. Best J. Wearable technology: covid-19 and the rise of remote clinical monitoring. BMJ 2021;372:n413.
131. Shubina V, Ometov A, Lohan ES. Technical Perspectives of Contact-Tracing Applications on Wearables for COVID-19 Control. 2020 12th International Congress on Ultra Modern Telecommunications and Control Systems and Workshops (ICUMT). Brno, Czech Republic; 2020.p. 229-35,
Cite This Article
How to Cite
Xiang, T.; Liu Z.; Ji N.; Lu L.; Clifton D. A.; Li X.; Deen M. J.; Lovell N. H.; Veetil J. C.; Zhu H.; Yan B.; Mok V.; Zhang Y. T. Towards wearable sensing-based precise and rapid responding system for the early detection of future pandemic. Conn. Health. Telemed. 2023, 2, 200007. http://dx.doi.org/10.20517/chatmed.2023.02
Download Citation
Export Citation File:
Type of Import
Tips on Downloading Citation
Citation Manager File Format
Type of Import
Direct Import: When the Direct Import option is selected (the default state), a dialogue box will give you the option to Save or Open the downloaded citation data. Choosing Open will either launch your citation manager or give you a choice of applications with which to use the metadata. The Save option saves the file locally for later use.
Indirect Import: When the Indirect Import option is selected, the metadata is displayed and may be copied and pasted as needed.
About This Article
Copyright
Data & Comments
Data
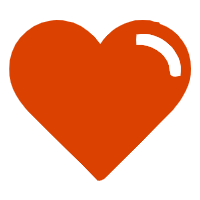
Comments
Comments must be written in English. Spam, offensive content, impersonation, and private information will not be permitted. If any comment is reported and identified as inappropriate content by OAE staff, the comment will be removed without notice. If you have any queries or need any help, please contact us at support@oaepublish.com.