Anti-angiogenic therapy in head and neck squamous cell carcinoma - current limitations and future directions
Abstract
Angiogenesis, the formation of new blood vessels, plays a crucial role in the progression and metastasis of various cancers, including head and neck squamous cell carcinoma (HNSCC). HNSCCs are characterized by altered levels of angiogenesis-related factors, including the overexpression of pro-angiogenic factors such as vascular endothelial growth factor (VEGF) and platelet-derived growth factor (PDGF), as well as the dysregulation of angiogenesis inhibitors. Together, these factors drive the formation of new blood vessels within the tumor microenvironment and are considered therapeutic targets in HNSCC. Although preclinical studies are promising, challenges have emerged in the clinical use of anti-angiogenic agents in the clinic, including treatment-related toxicities and the development of resistance to therapy. There is an unmet need for further research to elucidate the molecular pathways involved in HNSCC angiogenesis, identify novel therapeutic targets, and discover predictive biomarkers to improve patient selection.
Keywords
INTRODUCTION
Originating from the epithelial cells lining the upper aerodigestive tract, head and neck squamous cell carcinoma (HNSCC) is the sixth most common cancer worldwide[1-4]. HNSCC is a complex and multifactorial disease that accounts for approximately 890,000 new cases and 450,000 deaths annually[3,5]. The clinical appearance of the disease is often a painless lump or ulcer, which can be accompanied by difficulties in swallowing, hoarseness, or persistent cough[6]. Various sites can be affected in the head and neck region, including the oral cavity, pharynx, larynx, nasal cavity, and paranasal sinuses[1,4]. Therefore, the associated symptoms are heterogeneous and depend on the anatomical site as well as the etiology of the tumor[1]. The main risk factors for HNSCC include tobacco and alcohol abuse, betel nut chewing, and exposure to environmental pollutants[1,7]. Especially the combination of alcohol and tobacco consumption potentiates the risk of malignant development 35-fold in a synergistic manner[8]. Additionally, as a biologically distinct subgroup, infections with human papillomavirus (HPV) or Epstein-Barr virus (EBV) are significant risk factors[1,9].
Diagnosis of HNSCC requires clinical examination, including a detailed history and physical examination of the head and neck[10,11]. Further, to confirm the suspected clinical diagnosis, a histopathological work-up of a tumor biopsy is necessary, either obtained by surgical resection or by fine needle aspiration cytology[1,6,10,12]. Imaging studies such as computed tomography (CT), magnetic resonance imaging (MRI), and positron emission tomography (PET) are part of the staging process, determining the extent and invasiveness of the tumor as well as detecting metastasis and aiding in the estimation of the prognosis[5,6].
Treatment options for HNSCC depend on the stage and location of the tumor, as well as the patient's overall health[1]. The primary treatment options include surgery, radiation therapy, chemotherapy, and targeted therapy. Single-modality therapy is the preferred treatment for early-stage HNSCC with an overall better prognosis, while a multimodal treatment is typically used in advanced-stage disease[6]. A promising new approach to the treatment of HNSCC is targeted therapy, inter alia involving immunotherapy to target immune checkpoints or the tumor microenvironment, including the tumor vasculature. These targeted therapies were particularly effective in patients with a positive HPV status (HPV+) or in patients with recurrent or metastatic HNSCCs with defined molecular characteristics, including upregulation of immune checkpoint inhibitors and tumor-associated antigens or the presence of cancer driver mutations[1,9,13].
Despite those treatment advances, the prognosis for HNSCCs remains poor, with a five-year survival rate of approximately 60%, and has decreased only slightly over the last decades[1,7]. Patients with early-stage disease have a better prognosis than those with advanced-stage disease, and patients with HPV+ tumors have a better prognosis than those with HPV-negative (HPV-) tumors[14]. Prevention and early detection are crucial for improving the prognosis of HNSCC, as the survival rate declines rapidly with the increased tumor stage. Strategies for prevention include avoiding tobacco and alcohol use, practicing good oral hygiene, getting vaccinated against HPV, and avoiding exposure to environmental carcinogens[1]. Still, new treatment modalities are continuously being investigated and promising results from targeted therapy emphasize the potential of reducing current limitations in HNSCC therapy. Here, we will consider the potential of targeted therapies to improve the current survival rates of HNSCC with special emphasis on approaches targeting the tumor vasculature.
TUMOR ANGIOGENESIS IN HNSCC
Progressing tumors, analogous to normal tissues, require sustenance in the form of nutrients and oxygen, as well as the ability to evacuate metabolic wastes and carbon dioxide for sufficient growth. Therefore, an increased contribution and drainage of blood are necessary, which are met by the initiation and extension of tumor-associated neovasculature[15]. This induction of angiogenesis is one of the “Hallmarks of Cancer”, defined by Hanahan and Weinberg, probably a rate-limiting step in the development of solid tumors and is initiated within the hypoxic tumor microenvironment[15,16]. Hereby, tumor-associated hypoxia
Figure 1. Molecular mechanisms of angiogenesis: (A) sprouting angiogenesis, (B) intussusceptive angiogenesis, (C) coalescent angiogenesis, (D) vessel co-option, and (E) vasculogenic mimicry.
Sprouting angiogenesis refers to the mechanism of capillary vessel growth out of pre-existing ones through degradation of the extracellular matrix and the basement membrane surrounding the ECs. This subsequently allows ECs to invade and disintegrate the surrounding matrix, proliferate, form new immature blood vessels, and, therefore, develop a more extensive network for the nourishment of the growing tumor[20]. Vascular endothelial growth factor (VEGF) is a widely expressed angiogenic growth factor and plays a crucial role in sprouting angiogenesis, as well as angiogenesis in general, by inducing EC proliferation and migration, mainly through filopodia tip cell formation[21]. Hypoxia also represents a potent inducer of sprouting angiogenesis and reveals its potential for tumor promotion as the unbridled growth of cancer causes ubiquitous hypoxic conditions[22]. Besides sprouting angiogenesis, intussusceptive angiogenesis is also considered a VEGF-dependent mechanism, where angiogenesis is achieved by splitting a capillary into two independent microvessels[23]. Observed during growth and vascular remodeling of predominantly venous or capillary vascular networks, intussusceptive angiogenesis is a well-defined process of reshaping pre-existing networks by bisecting the lumen through an intussusceptive pillar. It has been reported that circulating progenitor cells are then integrated into the forming gaps in the vessel walls, consequently resulting in the formation of trans-vascular pillars without endothelial proliferation[22,24]. Interestingly, intussusceptive angiogenesis has been identified as a potential mechanism for resistance to anti-angiogenic drugs. It is considered compensatory angiogenesis in this regard, as the energy requirements are minor, and the process is fairly quick. Additionally, intussusceptive angiogenesis can build a vascular network specifically tailored to the local geometric and hemodynamic prerequisites, aggravating cancer growth and aggressiveness[24].
Further, described as the reverse of intussusception, coalescent angiogenesis describes remodeling an initial, hemodynamically ineffective vascular mesh structure into a hierarchical tree structure, providing efficient convective flow. Herein, preferential flow pathways evolve within initially isotropic capillary meshes, progressively enlarging through the coalescence of capillaries and elimination of internal tissue pillars, resulting in the regression of less perfused and the strengthening of preferred capillaries[25]. However, coalescent angiogenesis has only been identified in embryonic development. Therefore, it remains to be investigated in future studies whether those mechanisms have any impact on tumor angiogenesis[18].
Regarding the connection between angiogenesis and cancer, vessel co-option and vasculogenic mimicry are particularly important. Although the precise molecular mechanism involved in vessel co-option in cancer remains unclear, it refers to a non-angiogenic process where tumor cells hijack the abluminal surface of blood vessels as lead structures. Through those vascular courses, cancer cells obtain oxygen and nutrients by infiltrating the ambient microenvironment[26]. Vasculogenic mimicry is another type of non-angiogenic growth. Instead of attaching to blood vessels, tumor cells differentiate into EC-like cells and create matrix structures resembling vessels, which are perfused and provide nourishment to hypoxic tumor areas[27].
Despite those mechanisms of angiogenesis used by cancer to generate an abundant number of vessels, tumors are usually hypoxic and nutrient-deprived, leading to the production of even more pro-angiogenic factors and, therefore, creating a self-reinforcing vicious cycle of further tumor angiogenesis[28]. An explanation for this idiosyncrasy has been found in the abnormal structure and subsequent malfunction of tumor vasculature, presenting as leaky, tortuous, dilated, saccular, and having a haphazard pattern of interconnection[29]. Another contributing factor to this hostile milieu of hypoxia and malnutrition is the compression of these already weakened vessels via physical forces exerted by overabundant cells and escaping fluids due to the leakiness of tumor vessels, consequentially raising interstitial pressure[30,31]. This can cause fluctuations in the blood flow, resulting in an erratic distribution of oxygen, nutrients, immune cells, and drugs[31]. Further, high values of microvessel density (MVD), a product of the aforementioned continuous and self-reinforcing tumor angiogenesis, are considered to be an adverse prognostic factor in HNSCC, associated with shorter overall survival (OS) and progression-free survival (PFS), as well as higher rates of metastasis[32,33]. The latter can be explained by the poor architectural integrity of the vasculature, facilitating the invasion of tumor cells into nearby blood or lymphatic vessels. The increased permeability of these vessels and the presence of pro-angiogenic and chemotactic signals are additional mechanisms linking increased tumor angiogenesis to metastasis in HNSCC[34]. The hypoxic tumor microenvironment sustains this process via HIF-1α-dependent activation of immune suppressor cells[35-37], enabling escape from immune surveillance[38], as well as via expression of angiogenic factors by these immunosuppressive cells[39]. Additionally, hypoxia-induced and hypoxia-inducible factor 1 subunit α (HIF-1α)-mediated VEGF release from tumor cells is a strong contributor to immune suppression by preventing cytotoxic T-cell migration[40] and maturation of dendritic cells[41], thus linking angiogenesis to deprivation of immune response and ultimately leading to tumor progression.
Tumor angiogenesis involves a complicated interaction between ECs, cancer cells, immune cells, and the surrounding micro- and macro-environments. Many vascular and endothelial factors are at play, contributing to a complex chain of signaling mechanisms and cascades. All of these factors could serve as potential targets for therapy, complicating the search for the right starting point for anti-angiogenic therapies in HNSCC.
ANTI-ANGIOGENIC THERAPIES IN HNSCC
Targeting the VEGF pathway
In an effort to tackle tumor growth at its many routes, several therapeutic options are available specifically for targeting tumor angiogenesis. Four general categories with 14 different US Food and Drug Administration (FDA)-approved anti-angiogenic therapies have been established: ligand-directed antibodies, receptor-directed antibodies, small molecule inhibitors, and immunomodulatory agents[16]. The efficacy of these approaches in HNSCC is presented in Table 1 based on the available data from clinical trials. Similar findings in other malignant entities are summarized in Supplementary Table 1. VEGF was of particular interest in the ligand-directed and receptor-directed categories, as overexpression of VEGF is observed in HNSCC and is linked to worse OS[42,43]. Additionally, preclinical studies exploiting the anti-angiogenic effects of Bevacizumab, a monoclonal antibody targeting VEGF-A, showed enhanced tumor response in combination with radiation, resulting in reduced tumor blood vessel formation and inhibition of tumor growth[44]. Bevacizumab was initially approved by the FDA in 2004 as part of a combinational therapy for metastatic colorectal cancer[45]. Since then, five additional types of solid tumors have been approved for treatment with bevacizumab. However, the FDA has not yet approved anti-angiogenic agents for the treatment of HNSCC, despite the highly vascularized nature of the tissues in which HNSCC arises[46]. Argiris et al. evaluated the addition of bevacizumab to platinum-based chemotherapy in recurrent/metastatic HNSCC in their phase III randomized trial (NCT00588770)[47]. Although the median OS was improved from 11.0 months with chemotherapy alone to 12.6 months with chemotherapy and bevacizumab, those results were not significant (HR: 0.87; 95%CI: 0.70-1.09; P = 0.22). However, a significant increase in response rate, from 24.5% to 35.5% (P = 0.013), and PFS, from 4.3 months to 6.0 months (P = 0.0012), was observed. Those results were encouraging, even though bevacizumab treatment was associated with a significant increase in treatment-related toxicities. Consequently, further trials targeting VEGF were set up. Among others, Yoo et al. combined bevacizumab, erlotinib, a tyrosine kinase inhibitor (TKI) of the epithelial growth factor receptor (EGFR), and concurrent chemoradiation in locally advanced HNSCC (NCT00140556)[48]. Even though an increased risk of osteoradionecrosis was detected, complete response rates were achieved in 96% of patients (95%CI: 82%-100%) and 3-year OS and PFS reached 86% and 82%, respectively (95%CI: 66%-94% and 62%-92%)[49]. Cohen et al. conducted another phase I/II study (NCT00055913) where bevacizumab was combined with erlotinib in patients with recurrent/metastatic HNSCC[50]. Overall, the combination was well-tolerated with a median OS of 7.1 months (95%CI: 5.7-9.0 months) and PFS of 4.1 months (95%CI: 2.8-4.4 months), but the authors also conceded that the objective response of cetuximab/platinum/fluorouracil or other cytotoxic chemotherapy combinations in patients with recurrent or metastatic disease is likely to be higher than that noted in their study[49,50].
Categories of US Food and Drug Administration (FDA)-approved anti-angiogenic therapies: ligand-directed antibodies, receptor-directed antibodies, small molecule inhibitors, and immunomodulatory agents
Target of anti-angiogenic therapy | Ligand-directed antibodies | ||
Treatment modality | Bevacizumab, and chemotherapy or radiotherapy | ||
Clinical results in HNSCCs | Not approved by FDA in HNSCC Clinical trial: phase III in recurrent/metastatic HNSCC (NCT00588770) Results: significant increase in response rate/PFS[47] | ||
Target of anti-angiogenic therapy | Ligand-directed antibodies combined with receptor-directed antibodies | ||
Treatment modality | Bevacizumab and erlotinib, with or without chemotherapy | ||
Clinical results in HNSCCs | Clinical trial: locally advanced HNSCC (NCT00140556)[48] Results: complete response rates were achieved in 96% of patients, and 3-year OS and PFS reached 86% and 82%, respectively[49] Clinical trial: phase I/II in recurrent/metastatic HNSCC (NCT00055913) Results: combinations predispose to higher response[49,50] | ||
Target of anti-angiogenic therapy | Receptor-directed antibodies combined with immune checkpoint inhibitors | ||
Treatment modality | Ramucirumab, a monoclonal antibody targeting VEGFR2 and pembrolizumab, a monoclonal antibody, FDA-approved for treatment of HNSCC, against programmed cell death protein 1 (PD-1) | Apatinib, VEGFR2 inhibitor, and camrelizumab, anti-PD-1 monoclonal antibody | Bevacizumab and atezolizumab, anti-PD-1 monoclonal antibody |
Clinical results in HNSCCs | Clinical trial: one ongoing phase I/II in patients with recurrent/metastatic HNSCC (NCT03650764) Results: pending | Clinical trial: phase II in locally advanced resectable oral squamous cell carcinoma (OSCC) (NCT04393506) Results: major pathological response (MPR), defined as ≤ 10% residual viable tumor cells, in 40% of patients and 18-month locoregional recurrence and survival rates of 10.5% and 95%, respectively Treatment was well-tolerated, and the safety profile was manageable, superior to prior neoadjuvant chemotherapy[54] | Clinical trial: ongoing phase II in patients with recurrent or metastatic previously treated HNSCC (NCT03818061) Results: pending |
Target of anti-angiogenic therapy | Tyrosine kinase inhibitors | ||
Treatment modality | Sorafenib and/or sunitinib, targeting multiple receptors involved in angiogenesis, including VEGFR | ||
Clinical results in HNSCCs | Clinical trials: phase I and II in patients with recurrent or metastatic HNSCC Results: anticancer activity remained modest in combinatorial trials, single-agent use of those TKIs was not recommended[49] | ||
Target of anti-angiogenic therapy | Histone deacetylase inhibitors (HDACi) | ||
Treatment modality | Romidepsin - Zn-dependent histone deacetylase inhibitor | Vorinostat combined with chemotherapy and/or radiation therapy | Vorinostat combined with pembrolizumab |
Clinical results in HNSCCs | Clinical trial: phase II in patients with HNSCC patients (NCT00084682) Results: limited activity for the treatment of HNSCC. Objective responses were not observed, although 2 heavily pretreated patients had brief clinical disease stabilization[83] | Clinical trial: phase I trial in patients with stage III or stage IVa squamous cell cancer of the oropharynx which is either unresectable or borderline resectable (NCT01064921) Results: non-posted Clinical trial: phase II in patients with unresectable locally advanced (LA) oropharygeal (OP) squamous cell carcinoma (NCT01695122) Results: valproic acid promotes radiosensitization. VPA and CRT offered high RR; however, with prohibitive toxicities, which led to early trial termination | Clinical trial: phase II in resistant refractory solid tumors (NCT00404508) Results: a clinical benefit was observed in 12 (80%) patients: four PR, and eight SD[84] |
Synergistic effects of immunotherapy and anti-angiogenic therapy
Activation of pro-angiogenic pathways, especially mediated via the VEGF-VEGF receptor 2 (VEGFR2) interaction, strongly interferes with immune cell functions, leading to multi-target deprivation of immune response. This includes inhibition of immune cell differentiation, impaired antigen presentation, T cell exhaustion, and blockade of their infiltration. Concurrently, increasing expansion of regulatory T cells (Tregs), myeloid-derived suppressor cells (MDSCs), and tumor-associated macrophages (TAMs) at the tumor site was reported[51]. Thus, new combinatorial approaches targeting tumor angiogenesis and the tumor-associated immune response were developed and implemented in clinical trials.
Regarding VEGFR-directed therapy, the synergistic effects of immunotherapy and anti-angiogenic therapy, previously reported in non-small-cell lung cancer (NSCLC), are the aim of several ongoing trials involving ramucirumab, a monoclonal antibody targeting VEGFR2[52,53]. Ramucirumab gained regulatory approval in NSCLC, gastric cancer, colorectal cancer, and hepatocellular carcinoma[16]. There is one ongoing phase I/II clinical trial (NCT03650764) investigating ramucirumab plus pembrolizumab, a monoclonal antibody, FDA-approved for the treatment of HNSCC, against programmed cell death protein 1 (PD-1), in patients with recurrent/metastatic HNSCC. While results are still pending, the investigators hypothesize that simultaneous inhibition of angiogenesis and PD-1 will be more effective than inhibition of PD-1 alone, as a similar trial in patients with previously treated advanced NSCLC, gastro-oesophageal cancer, or urothelial carcinomas showed favorable antitumor activity under a manageable safety profile[53]. Additionally, targeting VEGFR2, Ju et al. investigated the VEGFR2 inhibitor, apatinib, in combination with anti-PD-1 camrelizumab in a neoadjuvant setting for locally advanced resectable oral squamous cell carcinoma (OSCC)[54]. In their pilot study (NCT04393506), 20 patients received three cycles of apatinib plus camrelizumab before surgery, which yielded a major pathological response (MPR), defined as ≤ 10% residual viable tumor cells, in 40% of their patients and 18-month locoregional recurrence and survival rates of 10.5% (95%CI: 0%-24.3%) and 95% (95%CI: 85.4%-100.0%), respectively. In addition to pathological efficacy, the treatment was well tolerated and the safety profile was manageable, even superior, to prior neoadjuvant chemotherapy regimens, showing no neoadjuvant therapy-related adverse effects of grade 3 or above[54]. The same promising concept of combining anti-angiogenic agents with immune checkpoint inhibitors is currently followed in the ongoing phase II study ATHENA (NCT03818061), combining atezolizumab, another monoclonal PD-1-antibody, and bevacizumab in patients with recurrent or metastatic previously treated HNSCC.
Anti-angiogenic tyrosine kinase inhibitors
Other key anti-angiogenic agents studied in HNSCC are sorafenib and sunitinib, the TKIs targeting multiple receptors involved in angiogenesis, including VEGFR. In phase I and II clinical trials, sorafenib and sunitinib were generally well-tolerated and demonstrated activity in patients with recurrent or metastatic HNSCC. However, this anticancer activity remained modest in combinatorial trials, and further studies with single-agent use of those TKIs were not recommended[49].
Histone deacetylase inhibitors
Histone deacetylases (HDACs) are involved in mediating multiple biological processes, including angiogenesis via the upregulation of HIF-1α, VEGF, and CXCR4[55]. Inhibitors of HDACs were approved by the FDA regarding hematological malignancies. However, the clinical efficacy in solid tumors is still being investigated[56]. Recent proteomic studies of HDAC inhibitor-resistant and sensitive cells suggested several potential drug combinations to overcome drug resistance in solid tumors[57]. Moreover, the treatment of cisplatin-resistant HNSCC cells with the class IIa HDAC inhibitor CHDI0039 resulted in promising preclinical results, unlike treatment with a class I/pan-HDAC inhibitor or combinations with bortezomib[58]. It was demonstrated in HNSCC that HDAC inhibitors inactivate ADP-ribosylation factor 1 (Arf1), which coordinates vesicle-mediated intracellular trafficking through degradation of epidermal growth factor receptor (EGFR), and thus inhibit invasion of tumor cells[59]. Clinical trials involving the use of HDAC inhibitors in HNSCC are presented in Table 1.
Resistance to anti-angiogenic therapies
One contributing factor to the limited efficacy of anti-angiogenic therapy seems to be drug resistance over time[60]. In this regard, extracellular vesicles (EVs) and the aforementioned vessel co-option have been observed to play an essential part in resistance to anti-angiogenic therapy, presumably due to their non-angiogenic nature[60,61]. EVs are naturally occurring nano-sized membrane-bound vesicles released by nearly all cell types and represent critical mediators of intercellular communication, especially between tumor and stromal cells[62-65]. A growing body of evidence suggests that tumor cell-derived EVs promote tumor angiogenesis in two ways: directly transferring bioactive cargos to ECs and exerting pro-angiogenic effects through other cells like fibroblasts and immune cells, doubling the potential for resistance[60,64,66,67]. While the exact pathophysiological mechanisms of vessel co-option are still unclear, as indicated above, studies in colorectal cancer have shown that treatment with bevacizumab can be less effective in cases where vessel co-option is present, suggesting that vessel co-option may play a role in resistance to anti-angiogenic therapy[61,68,69].
A potential method to overcome resistance to anti-angiogenic therapies is the usage of vascular disrupting agents (VDAs), such as OXi4503, combretastatin A4 phosphate (CA4P), or ombrabulin. Immature tumor vessels were shown to be more sensitive to VDAs[70]. However, the heterogeneity within the tumor microenvironment with regard to molecular and morphological differences was suspected as a major contributor to VDA treatment resistance[71]. The combination of VDAs and anti-angiogenic agents was investigated and promised to enhance the response to anti-angiogenic therapies; however, cumulative toxicities were identified as a major challenge[72-74].
Histological biomarkers for anti-angiogenic therapies
A connection between angiogenesis and HNSCC tumorigenesis, as well as metastasis, was already presented decades ago, predominantly by assessing expression levels of VEGF in tumor tissues[75,76]. However, the successful clinical implementation of anti-angiogenic therapies in HNSCC will rely on further identification of adequate diagnostic and prognostic biomarkers. Hereby, markers of the angiogenic switch in HNSCC, related to both endothelial cell and hypoxic signaling, were suggested as angiogenic biomarkers. These markers were further divided into diagnostic and prognostic biomarkers. Galectin-1, Galectin-3; p21, Cyclin D1; FGFR1, and FGFR3 were described as the most promising diagnostic biomarkers, while CD31 and CD34 are accurate indicators of MVD and D240 of lymphatic vessel density in HNSCC. LOXL-2 is a participant in ECM remodeling; VEGFR-3, CCR7, NRP1, and SEMA3E are associated with lymph node metastasis; carbonic anhydrase-9 (CA-9), HIF-1α, or HSP70 are related to hypoxia[77]. Furthermore, vascular cell adhesion molecule-1 (VCAM-1) and intercellular adhesion molecule-1 (ICAM-1) are noteworthy biomarkers, as they are both contributors to tumor angiogenesis and possess predictive value in cancer but have not been sufficiently studied in HNSCC, yet[78-80]. On the one hand, upregulation of VCAM-1 expression in the tumor periphery can generally initiate angiogenesis via very late antigen-4 (VLA-4) expressed on vascular stem cells and progenitor cells, leading to a cell-homing process to VCAM-1 in the tumor environment[81]. On the other hand, regarding VCAM-1 and ICAM-1 expression on endothelial cells of the tumor vasculature, a downregulation has been shown to play a crucial role in protecting the tumor by inhibiting leukocyte infiltration and, therefore, reducing the local immune response, which has been observed in HNSCC[82]. Interestingly, the aforementioned downregulation of VCAM-1 is directly associated with VEGF expression levels[78]. This inconsistency in expression levels of adhesion molecules, in regard to the effect it has on the tumor depending on location, highlights a site-specific interaction between the vasculature and its immediate surroundings and requires further investigation into these crosstalks in HNSCC and cancer in general[79]. Subsequently, establishing biological markers for angiogenesis in HNSCC promises to help implement tailored anti-angiogenic therapies analogous to currently widely used markers such as EGFR.
CONCLUSION
Anti-angiogenic therapies used in preclinical studies have shown to be effective in treating HNSCC, whereas, in clinical trials, challenges and limitations are frequently observed. One challenge is selecting patients who are most likely to benefit from anti-angiogenic treatments, as in certain therapy combinations, only some patients seem to derive sustained benefit and complete responses. In contrast, other patients do not benefit from anti-angiogenic therapies, but experience increased treatment-associated toxicities. Another challenge is the emergence of resistance to the selected anti-angiogenic therapies, as tumors may pursue alternative angiogenic pathways, rendering the anti-angiogenic treatments ineffective. Clearly, further studies of angiogenic mechanisms in cancer and of resistance to anti-angiogenic therapies are required to establish which, when, and in what combinations anti-angiogenic agents should be used in HNSCC. The one unmet and urgent need that could make a difference and lead to improved outcomes is the development of predictive biomarkers of response to therapy. Such biomarkers could guide selections of drugs and treatments and serve as disease-specific monitors of cancer progression. Currently, intensive efforts are invested in examining circulating tumor cells (CTC), circulating tumor DNA (ctDNA), and tumor-derived small EVs for their potential to serve as liquid tumor biopsies.
DECLARATIONS
Authors’ contributions
Writing of the manuscript: Nieberle F, Spoerl S, Ludwig N
Supervision and editing of the manuscript: Głuszko A, Taxis J, Spanier G, Erber R, Spoerl S, Szczepański MJ, Beckhove P, Reichert TE, Whiteside TL, Ludwig N
Availability of data and materials
Not applicable.
Financial support and sponsorship
Szczepański MJ was supported by National Science Centre, Poland UMO-2017/26/M/NZ5/00877#. Whiteside TL was partially supported by NIH grants U01-DE029759, R01-DE031299, and R01-CA256068. Ludwig N was supported by the Walter Schulz Foundation and the Helga-Reifert-Foundation.
Conflicts of interest
All authors declared that there are no conflicts of interest.
Ethical approval and consent to participate
Not applicable.
Consent for publication
Not applicable.
Copyright
© The Author(s) 2024.
Supplementary Materials
REFERENCES
1. Johnson DE, Burtness B, Leemans CR, Lui VWY, Bauman JE, Grandis JR. Head and neck squamous cell carcinoma. Nat Rev Dis Primers 2020;6:92.
2. Ferlay J, Colombet M, Soerjomataram I, et al. Estimating the global cancer incidence and mortality in 2018: GLOBOCAN sources and methods. Int J Cancer 2019;144:1941-53.
3. Bray F, Ferlay J, Soerjomataram I, Siegel RL, Torre LA, Jemal A. Global cancer statistics 2018: GLOBOCAN estimates of incidence and mortality worldwide for 36 cancers in 185 countries. CA A Cancer J Clin 2018;68:394-424.
4. Curry JM, Sprandio J, Cognetti D, et al. Tumor microenvironment in head and neck squamous cell carcinoma. Semin Oncol 2014;41:217-34.
6. Ernani V, Saba NF. Oral cavity cancer: risk factors, pathology, and management. Oncology 2015;89:187-95.
7. Brouwer AF, He K, Chinn SB, et al. Time-varying survival effects for squamous cell carcinomas at oropharyngeal and nonoropharyngeal head and neck sites in the United States, 1973-2015. Cancer 2020;126:5137-46.
8. Hashibe M, Brennan P, Chuang S, et al. Interaction between tobacco and alcohol use and the risk of head and neck cancer: pooled analysis in the international head and neck cancer epidemiology consortium. Cancer Epidemiol Biomarkers Prev 2009;18:541-50.
9. von Witzleben A, Wang C, Laban S, Savelyeva N, Ottensmeier CH. HNSCC: tumour antigens and their targeting by immunotherapy. Cells 2020;9:2103.
10. Chamoli A, Gosavi AS, Shirwadkar UP, et al. Overview of oral cavity squamous cell carcinoma: risk factors, mechanisms, and diagnostics. Oral Oncol 2021;121:105451.
11. Walsh T, Liu JL, Brocklehurst P, et al. Clinical assessment to screen for the detection of oral cavity cancer and potentially malignant disorders in apparently healthy adults. Cochrane Database Syst Rev 2013;2013:Cd010173.
12. Fitzhugh VA, Maniar KP, Gurudutt VV, Rivera M, Chen H, Wu M. Fine-needle aspiration biopsy of granular cell tumor of the tongue: a technique for the aspiration of oral lesions. Diagn Cytopathol 2009;37:839-42.
13. Wang H, Zhao Q, Zhang Y, et al. Immunotherapy advances in locally advanced and recurrent/metastatic head and neck squamous cell carcinoma and its relationship with human papillomavirus. Front Immunol 2021;12:652054.
14. Chaturvedi AK, Engels EA, Pfeiffer RM, et al. Human papillomavirus and rising oropharyngeal cancer incidence in the United States. J Clin Oncol 2011;29:4294-301.
16. Micaily I, Johnson J, Argiris A. An update on angiogenesis targeting in head and neck squamous cell carcinoma. Cancers Head Neck 2020;5:5.
17. Schito L. Bridging angiogenesis and immune evasion in the hypoxic tumor microenvironment. Am J Physiol Regul Integr Comp Physiol 2018;315:R1072-84.
18. Dudley AC, Griffioen AW. Pathological angiogenesis: mechanisms and therapeutic strategies. Angiogenesis 2023;26:313-47.
19. Udan RS, Culver JC, Dickinson ME. Understanding vascular development. Wiley Interdiscip Rev Dev Biol 2013;2:327-46.
20. Hillen F, Griffioen AW. Tumour vascularization: sprouting angiogenesis and beyond. Cancer Metastasis Rev 2007;26:489-502.
21. Gerhardt H, Golding M, Fruttiger M, et al. VEGF guides angiogenic sprouting utilizing endothelial tip cell filopodia. J Cell Biol 2003;161:1163-77.
22. Ackermann M, Werlein C, Plucinski E, et al. The role of vasculature and angiogenesis in respiratory diseases. Angiogenesis 2024:1-18.
23. Baum O, Suter F, Gerber B, et al. VEGF-A promotes intussusceptive angiogenesis in the developing chicken chorioallantoic membrane. Microcirculation 2010;17:447-57.
24. Saravanan S, Vimalraj S, Pavani K, Nikarika R, Sumantran VN. Intussusceptive angiogenesis as a key therapeutic target for cancer therapy. Life Sci 2020;252:117670.
25. Nitzsche B, Rong WW, Goede A, et al. Coalescent angiogenesis-evidence for a novel concept of vascular network maturation. Angiogenesis 2022;25:35-45.
26. Zhang Y, Wang S, Dudley AC. Models and molecular mechanisms of blood vessel co-option by cancer cells. Angiogenesis 2020;23:17-25.
27. Wagenblast E, Soto M, Gutiérrez-ángel S, et al. A model of breast cancer heterogeneity reveals vascular mimicry as a driver of metastasis. Nature 2015;520:358-62.
28. Carmeliet P, Jain RK. Principles and mechanisms of vessel normalization for cancer and other angiogenic diseases. Nat Rev Drug Discov 2011;10:417-27.
29. Jain RK. Normalization of tumor vasculature: an emerging concept in antiangiogenic therapy. Science 2005;307:58-62.
30. Datta M, Coussens LM, Nishikawa H, Hodi FS, Jain RK. Reprogramming the tumor microenvironment to improve immunotherapy: emerging strategies and combination therapies. Am Soc Clin Oncol Educ Book 2019;39:165-74.
31. Carmeliet P, Jain RK. Molecular mechanisms and clinical applications of angiogenesis. Nature 2011;473:298-307.
32. Dong Y, Ma G, Liu Y, Lu S, Liu L. Prognostic value of microvessel density in head and neck squamous cell carcinoma: a meta-analysis. Dis Markers 2020;2020:1-11.
33. Evans M, Baddour HM Jr, Magliocca KR, et al. Prognostic implications of peritumoral vasculature in head and neck cancer. Cancer Med 2019;8:147-54.
34. Bielenberg DR, Zetter BR. The contribution of angiogenesis to the process of metastasis. Cancer J 2015;21:267-73.
35. Clambey ET, Mcnamee EN, Westrich JA, et al. Hypoxia-inducible factor-1 alpha-dependent induction of FoxP3 drives regulatory T-cell abundance and function during inflammatory hypoxia of the mucosa. Proc Natl Acad Sci USA 2012;109:E2784-93.
36. Corzo CA, Condamine T, Lu L, et al. HIF-1α regulates function and differentiation of myeloid-derived suppressor cells in the tumor microenvironment. J Exp Med 2010;207:2439-53.
37. Doedens AL, Stockmann C, Rubinstein MP, et al. Macrophage expression of hypoxia-inducible factor-1α suppresses T-cell function and promotes tumor progression. Cancer Res 2010;70:7465-75.
38. Zhang H, Lu H, Xiang L, et al. HIF-1 regulates CD47 expression in breast cancer cells to promote evasion of phagocytosis and maintenance of cancer stem cells. Proc Natl Acad Sci USA 2015;112:E6215-23.
39. Burke B, Tang N, Corke KP, et al. Expression of HIF-1α by human macrophages: implications for the use of macrophages in hypoxia-regulated cancer gene therapy. J Pathol 2002;196:204-12.
40. Ohm JE, Gabrilovich DI, Sempowski GD, et al. VEGF inhibits T-cell development and may contribute to tumor-induced immune suppression. Blood 2003;101:4878-86.
41. Gabrilovich DI, Chen HL, Girgis KR, et al. Production of vascular endothelial growth factor by human tumors inhibits the functional maturation of dendritic cells. Nat Med 1996;2:1096-103.
42. Zang J, Li C, Zhao L, et al. Prognostic value of vascular endothelial growth factor in patients with head and neck cancer: a meta-analysis. Head Neck 2013;35:1507-14.
43. Kyzas PA, Cunha IW, Ioannidis JP. Prognostic significance of vascular endothelial growth factor immunohistochemical expression in head and neck squamous cell carcinoma: a meta-analysis. Clin Cancer Res 2005;11:1434-40.
44. Hoang T, Huang S, Armstrong E, Eickhoff JC, Harari PM. Enhancement of radiation response with bevacizumab. J Exp Clin Cancer Res 2012;31:37.
45. Shih T, Lindley C. Bevacizumab: an angiogenesis inhibitor for the treatment of solid malignancies. Clin Ther 2006;28:1779-802.
46. Salem A, Hadler-olsen E, Al-samadi A. Editorial: angiogenesis and angiogenesis inhibitors in oral cancer. Front Oral Health 2021;2:816963.
47. Argiris A, Li S, Savvides P, et al. Phase III randomized trial of chemotherapy with or without bevacizumab in patients with recurrent or metastatic head and neck cancer. J Clin Oncol 2019;37:3266-74.
48. Yoo DS, Kirkpatrick JP, Craciunescu O, et al. Prospective trial of synchronous bevacizumab, erlotinib, and concurrent chemoradiation in locally advanced head and neck cancer. Clin Cancer Res 2012;18:1404-14.
49. Hyytiäinen A, Wahbi W, Väyrynen O, et al. Angiogenesis inhibitors for head and neck squamous cell carcinoma treatment: is there still hope? Front Oncol 2021;11:683570.
50. Cohen EE, Davis DW, Karrison TG, et al. Erlotinib and bevacizumab in patients with recurrent or metastatic squamous-cell carcinoma of the head and neck: a phase I/II study. Lancet Oncol 2009;10:247-57.
51. Rahma OE, Hodi FS. The intersection between tumor angiogenesis and immune suppression. Clin Cancer Res 2019;25:5449-57.
52. Yao J, Wang Z, Sheng J, et al. Efficacy and safety of combined immunotherapy and antiangiogenic therapy for advanced non-small cell lung cancer: a two-center retrospective study. Int Immunopharmacol 2020;89:107033.
53. Herbst RS, Arkenau H, Santana-davila R, et al. Ramucirumab plus pembrolizumab in patients with previously treated advanced non-small-cell lung cancer, gastro-oesophageal cancer, or urothelial carcinomas (JVDF): a multicohort, non-randomised, open-label, phase 1a/b trial. Lancet Oncol 2019;20:1109-23.
54. Ju W, Xia R, Zhu D, et al. A pilot study of neoadjuvant combination of anti-PD-1 camrelizumab and VEGFR2 inhibitor apatinib for locally advanced resectable oral squamous cell carcinoma. Nat Commun 2022;13:5378.
55. Liang D, Kong X, Sang N. Effects of histone deacetylase inhibitors on HIF-1. Cell Cycle 2006;5:2430-5.
56. Liang T, Wang F, Elhassan RM, et al. Targeting histone deacetylases for cancer therapy: trends and challenges. Acta Pharmaceutica Sinica B 2023;13:2425-63.
57. Hao B, Ma K, Xu J, et al. Proteomics analysis of histone deacetylase inhibitor-resistant solid tumors reveals resistant signatures and potential drug combinations. Acta Pharmacol Sin 2024;45:1305-15.
58. Schrenk C, Bollmann LM, Haist C, et al. Synergistic interaction of the class IIa HDAC inhibitor CHDI0039 with bortezomib in head and neck cancer cells. IJMS 2023;24:5553.
59. He L, Gao L, Shay C, Lang L, Lv F, Teng Y. Histone deacetylase inhibitors suppress aggressiveness of head and neck squamous cell carcinoma via histone acetylation-independent blockade of the EGFR-Arf1 axis. J Exp Clin Cancer Res 2019;38:84.
60. Ye Z, Yu Z, Chen G, Jia J. Extracellular vesicles in tumor angiogenesis and resistance to anti-angiogenic therapy. Cancer Sci 2023;114:2739-49.
61. Kuczynski EA, Reynolds AR. Vessel co-option and resistance to anti-angiogenic therapy. Angiogenesis 2020;23:55-74.
62. Becker A, Thakur BK, Weiss JM, Kim HS, Peinado H, Lyden D. Extracellular vesicles in cancer: cell-to-cell mediators of metastasis. Cancer Cell 2016;30:836-48.
63. Yu Z, Liu X, Wu M, et al. Untouched isolation enables targeted functional analysis of tumour-cell-derived extracellular vesicles from tumour tissues. J Extracell Vesicle 2022;11:e12214.
64. Ludwig N, Yerneni SS, Azambuja JH, et al. TGFβ+ small extracellular vesicles from head and neck squamous cell carcinoma cells reprogram macrophages towards a pro-angiogenic phenotype. J Extracell Vesicle 2022;11:12294.
65. Ludwig N, Yerneni SS, Harasymczuk M, et al. TGFβ carrying exosomes in plasma: potential biomarkers of cancer progression in patients with head and neck squamous cell carcinoma. Br J Cancer 2023;128:1733-41.
66. Ludwig N, Jackson EK, Whiteside TL. Role of exosome-associated adenosine in promoting angiogenesis. Vessel Plus 2020;4:8.
67. Ludwig N, Yerneni SS, Azambuja JH, et al. Tumor-derived exosomes promote angiogenesis via adenosine A2B receptor signaling. Angiogenesis 2020;23:599-610.
68. Jeong H, Jones D, Liao S, et al. Investigation of the lack of angiogenesis in the formation of lymph node metastases. J Natl Cancer Inst 2015;107:djv155.
69. Frentzas S, Simoneau E, Bridgeman VL, et al. Vessel co-option mediates resistance to anti-angiogenic therapy in liver metastases. Nat Med 2016;22:1294-302.
70. Chan LS, Daruwalla J, Christophi C. Selective targeting of the tumour vasculature*. ANZ J Surg 2008;78:955-67.
71. Nguyen L, Fifis T, Malcontenti-wilson C, et al. Spatial morphological and molecular differences within solid tumors may contribute to the failure of vascular disruptive agent treatments. BMC Cancer 2012;12:522.
72. Nguyen L, Fifis T, Christophi C. Vascular disruptive agent OXi4503 and anti-angiogenic agent Sunitinib combination treatment prolong survival of mice with CRC liver metastasis. BMC Cancer 2016;16:533.
73. Garcia V, Basu B, Molife LR, Kaye SB. Combining antiangiogenics to overcome resistance: rationale and clinical experience. Clin Cancer Res 2012;18:3750-61.
74. Siemann DW, Shi W. Efficacy of combined antiangiogenic and vascular disrupting agents in treatment of solid tumors. Int J Rad Oncol Biol Phys 2004;60:1233-40.
75. Sauter ER, Nesbit M, Watson JC, Klein-Szanto A, Litwin S, Herlyn M. Vascular endothelial growth factor is a marker of tumor invasion and metastasis in squamous cell carcinomas of the head and neck. Clin Cancer Res 1999;5:775-82. Available from: https://aacrjournals.org/clincancerres/article/5/4/775/287485/Vascular [Last accessed on 20 Jun 2024].
76. Smith BD, Smith GL, Carter D, Sasaki CT, Haffty BG. Prognostic significance of vascular endothelial growth factor protein levels in oral and oropharyngeal squamous cell carcinoma. J Clin Oncol 2000;18:2046-52.
77. Alessandrini L, Astolfi L, Daloiso A, et al. Diagnostic, prognostic, and therapeutic role for angiogenesis markers in head and neck squamous cell carcinoma: a narrative review. Int J Mol Sci 2023;24:10733.
78. Schlesinger M, Bendas G. Vascular cell adhesion molecule-1 (VCAM-1)-An increasing insight into its role in tumorigenicity and metastasis. Int J Cancer 2015;136:2504-14.
79. Ebeling S, Kowalczyk A, Perez-vazquez D, Mattiola I. Regulation of tumor angiogenesis by the crosstalk between innate immunity and endothelial cells. Front Oncol 2023;13:1171794.
80. Chandler KB, Costello CE, Rahimi N. Glycosylation in the tumor microenvironment: implications for tumor angiogenesis and metastasis. Cells 2019;8:544.
81. Vanheyst KA, Choi SH, Kingsley DT, Huang AY. Ectopic tumor VCAM-1 expression in cancer metastasis and therapy resistance. Cells 2022;11:3922.
82. Lieder AM, Prior TG, Wood KJ, Werner JA. The relevance of adhesion molecules in the classification of squamous cell carcinoma of the head and neck. Anticancer Res 2005;25:4141-7.
83. Haigentz M, Kim M, Sarta C, et al. Phase II trial of the histone deacetylase inhibitor romidepsin in patients with recurrent/metastatic head and neck cancer. Oral Oncol 2012;48:1281-8.
Cite This Article

How to Cite
Download Citation
Export Citation File:
Type of Import
Tips on Downloading Citation
Citation Manager File Format
Type of Import
Direct Import: When the Direct Import option is selected (the default state), a dialogue box will give you the option to Save or Open the downloaded citation data. Choosing Open will either launch your citation manager or give you a choice of applications with which to use the metadata. The Save option saves the file locally for later use.
Indirect Import: When the Indirect Import option is selected, the metadata is displayed and may be copied and pasted as needed.
About This Article
Copyright
Data & Comments
Data
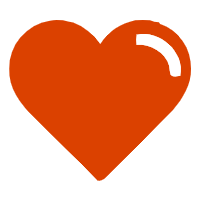
Comments
Comments must be written in English. Spam, offensive content, impersonation, and private information will not be permitted. If any comment is reported and identified as inappropriate content by OAE staff, the comment will be removed without notice. If you have any queries or need any help, please contact us at [email protected].