Severely calcified coronary artery lesions: focus on interventional management
Abstract
Severe coronary artery calcifications remain a challenge for the contemporary interventional cardiologist in the light of the growing demand for diagnostic procedures and interventions in elderly patients; in addition, the general prognostic improvement after percutaneous coronary intervention (PCI) is expanding the indications to PCI to increasingly complex anatomies. In the last decade, a renewed interest in the treatment of calcific lesions has been observed, with the aim to optimize the mechanic effects of balloon angioplasty and the expansion and apposition of DES to the vessel wall. However, patients with calcific coronary artery disease represent a subset with a high risk of adverse outcomes, both intra-procedural and in the long-term. The need to guarantee a targeted and tailored treatment based on the coronary anatomy of any individual patient is a current priority of the interventional community. The efficacy of rotational atherectomy in improving procedural success for the treatment of calcified lesions has been widely demonstrated. The advent of new technologies -especially of intravascular lithotripsy (IVL)-, the application of techniques and materials initially developed for as complex procedures as chronic total occlusions (CTO), the increasing experience of contemporary operators and the introduction of latest generation drug-eluting stents (DES) with excellent technical and structural properties, are further contributing to improving outcomes of current PCI for calcific lesions.
Keywords
INTRODUCTION
Severe coronary artery calcifications (CAC), already identified by Andreas Grüntzig as one of the main limitations to the feasibility of coronary balloon angioplasty[1], are a challenge for the contemporary interventional cardiologist as well for several reasons: first, the growing demand for diagnostic procedures and interventions in elderly patients who carry CAC because of aging; in addition, the general prognostic improvement after percutaneous coronary intervention (PCI) and the reduction in the incidence of restenosis with latest generation drug-eluting stents (DES) are expanding the indications to PCI to increasingly complex anatomies[2]. In the last decade, the renewed interest in tools for ablation or modification of calcified plaques paved the way to the development of new technologies and to technical and procedural improvements of those already existing, with the ultimate aim to optimize the mechanic effects of balloon angioplasty and the expansion and apposition of DES to the vessel wall.
EPIDEMIOLOGY AND PROGNOSTIC ROLE OF CORONARY ARTERY CALCIFICATIONS
All patients with cardiovascular disease virtually have a certain amount of CAC. Therefore, the assessment of CAC provides additional prognostic information over traditional risk factor-based assessment in predicting mortality risk[3]. The presence of CAC is epidemiologically associated with a higher cardiovascular risk profile[4], while prognostically, they are independent predictors of stent thrombosis and target vessel revascularization one year after PCI[5]. In an individual analysis of approximately 6,300 patients from 7 randomized trials of DES, it was demonstrated that the prevalence of severely calcified coronary lesions is associated with a higher incidence of incomplete revascularization and higher mortality[6]. Moreover, in a more recent analysis of 19,833 patients from 18 randomized trials, the negative prognostic impact of coronary artery calcification remained evident across a 5-year follow-up, an effect that was only mitigated by second-generation DES[7]. Therefore, calcified coronary lesions, in addition to being particularly frequent in contemporary practice, represent a negative prognostic indicator regardless of the clinical presentation or the type of DES implanted[8,9]. These negative outcomes are related not only to comorbidities but also to the greater technical complexity of PCI in calcified lesions, which represent an obstacle to the progression of stents through the coronary arteries and, in any case, reduce their expansion capacity; CAC can indeed damage the DES polymer and consequently alter the normal distribution kinetics and the uniformity of release of the anti-proliferative drug[10,11]. This results in an often-suboptimal procedural result and an increased risk of complications such as intrastent restenosis or stent thrombosis. Beyond this, CAC also increases the risk of acute procedural complications such as stent loss, dissection, and coronary artery perforation[12].
BIOLOGY AND HISTOLOGY OF CORONARY CALCIFICATIONS
Vascular calcification is an active biological process that reflects systemic inflammation finely regulated by cellular interaction mechanisms[13]. Histologically, vascular calcifications can be localized both in the tunica intima, representing a component of the atherosclerotic plaque ultimately responsible for vascular events, and in the tunica media, where they assume a more often circumferential appearance linked to the presence of elastic tissue and where they cause an increase in stiffness and decrease vascular compliance[14]. Overall, intimal calcifications are a dynamic phenomenon that can be considered as the barometer of the atherosclerotic process[15]: during the early stages of intimal thickening, calcified spots are associated with plaque instability, while in more advanced fibrocalcific lesions, confluent regions of calcification are observed with calcified sheets or plates where collagen matrix and necrotic core itself are calcified and associated with more stable atherosclerosis phenotypes, representing a final response to atherosclerotic damage[16]. Fracture in calcified sheets can lead to the formation of nodular calcification. Such nodules may prompt discontinuation of the endothelial lining, thus extending into the lumen, and promoting fibrin deposition and acute luminal thrombosis[15]. In pathological studies, calcified nodules are the underlying mechanism in 2% to 7% of coronary thrombosis[15]. Importantly, different types of calcified nodules can be identified at either pathology or intravascular imaging, such as eruptive and non-eruptive calcific nodules, and their management remains a matter of debate[17]. Calcifications of the tunica media are very common in patients with chronic kidney disease, especially in vessels typically spared by atherosclerosis, and they are often not associated with the narrowing of the vessel lumen[18]. The microscopic appearance of calcifications can be amorphous, i.e., devoid of any tissue architecture, but can even assume a chondro-osseous architecture, which presupposes a phenotypic change of the vascular smooth muscle cells into mesenchymal stem cells[16]. After DES implantation in calcified lesions, the same mechanisms, such as calcium sheet formation and reformation of calcified nodules inside DES, may be involved in DES failure.
EVALUATION OF CORONARY CALCIFICATIONS
Coronary calcifications can be detected by various diagnostic methods, in particular computed tomography (CT), angiography and intravascular imaging techniques. Intravascular ultrasound (IVUS) and optical coherence tomography (OCT) are the most used techniques. They allow a very accurate assessment of the calcium burden and its distribution and eccentricity, information that allows planning an individualized strategy for lesion preparation and optimization of stent implantation.
Coronary computed tomography angiography
CT is the most sensitive method for detecting coronary calcium[19]. It is mainly used in elective conditions to stratify medium and long-term cardiovascular risk through the calcium score (volumetric quantification of calcium) of the coronary arteries[20]. Apart from the general prognostic data, there are no currently established criteria for planning and guiding a specific interventional treatment based on the CT scan. A Korean group has proposed a new “calcification remodeling index” based on coronary CT scans, in addition to better known criteria such as calcium score, calcification volume or the number of quadrants involved in the calcification arc, to predict the use of rotational atherectomy during PCI[21].
Coronary angiography
Angiography is highly specific but lacks sensitivity compared with coronary CT and intravascular imaging for identifying coronary calcium. In the pivotal 1995 study by Gary Mintz, coronary angiography was indeed able to identify calcium in only 38% of cases, and sensitivity was even less for mild levels of calcification[22]. CAC are angiographically identified as linear radiopaque areas that follow the contour of the coronary artery in a synchronous motion with cardiac contraction prior to opacification with contrast [Figure 1]. They are defined as severe when both sides of the arterial wall are identified during cardiac movement in the absence of contrast dye[23]. It has been observed that those coronary calcifications that are not visible angiographically, and therefore can be identified only with IVUS or OCT, do not appear to limit stent expansion[24]. Consequently, the presence of angiographically visible calcium, i.e., a thick calcification, is likely to remain a good predictor of incomplete stent expansion. During contrast injection, calcium appears as a blurred area with inhomogeneous contrast opacity (haziness). Thus, differentiation between calcification and intraluminal thrombus can be difficult by angiography alone because severe CAC can appear as a lumen-filling defect, much like a thrombus that impedes the passage of contrast agent; hence, coronary angiography remains a suboptimal and scarcely accurate technique to identify high calcium content.
Figure 1. Angiographic demonstration of severe calcification of the left anterior descending artery (LAD). Two linear radio-opaque areas along either side of the LAD profile (A) can be observed prior to (B) contrast dye injection. Stent-enhancement features of the angiographic machine facilitate the identification of calcifications (C) after wiring and positioning a balloon with two radio-opaque markers.
Intravascular ultrasound
A heavily calcified lesion appears at IVUS as a hyperechoic arch associated with an acoustic shadow towards the periphery of the image, which makes further details in the vessel wall invisible [Figure 2]. In fact, ultrasounds have a spatial resolution of 150-200 μm but cannot propagate beyond the calcification since the calcium itself causes its total reflection towards the source. In a pioneering post-mortem study, IVUS reported 100% specificity and 90% sensitivity for identifying dense calcified plaques or clusters of micro-calcifications with much less ability to identify isolated micro-calcifications (< 50 μm) that remain below its spatial resolution[25]. Subsequent in vivo clinical data confirmed the greater sensitivity of IVUS in detecting coronary calcium compared with angiography (73% of cases vs. 38%; P < 0.001)[22]. The level of calcification can be quantified on IVUS measuring (in degrees) the circumference of the uninterrupted calcific arch and its length (measured in mm), and is classified as “superficial”, therefore close to or projecting into the lumen, or “deep”, with less impact on the profile of the vascular lumen. The measurement of the circumference arc has a consolidated value, as it is known that a superficial calcification > 180° configures a condition of greater risk of under-expansion of the stent[26]. In a recent study including de novo lesions undergoing DES implantation without pre-treatment with debulking devices, a score based on (1) superficial calcium angle > 270° longer than 5 mm; (2) 360° of superficial calcium; (3) calcific nodule; and (4) vessel diameter < 3.5 mm permitted to identify calcified plaques at risk for stent under-expansion and thus requiring adjunctive calcium modification techniques for lesion preparation[27]. In clinical practice, the coronary lesion can narrow the coronary lumen so much that it cannot be crossed by the IVUS catheter. This already represents a gross indication of marked coronary calcification and should readily indicate PCI with targeted calcium ablation techniques.
Figure 2. Intravascular ultrasound (IVUS) and optical coherence tomography (OCT) demonstration of coronary calcifications. In (A), an echodense image is observed on IVUS consistent with intimal calcification extending for about 2 quadrants, from 6 to 10 o’clock. In (B), a calcified ring is evident along the entire vessel circumference. In (C), a “signal-poor” image with well-defined margins is evident on OCT from 1 to 4 o’clock (asterisk), consistent with superficial calcifications. In (D), a voluminous calcific nodule is observed (asterisk) protruding into the vessel from 10 to 1 o’clock.
Optical coherence tomography
OCT is an extremely sensitive and specific intravascular method for detecting calcium; it is based on the backscattering of a light beam and guarantees a spatial resolution of about 10-20 μm. On OCT images [Figure 2], calcifications appear as well-defined areas of light-signal attenuation (signal-poor areas) but with a clear delineation of both luminal and extraluminal borders[28]. Due to its higher longitudinal and axial spatial resolution, OCT can overcome many of the IVUS limitations so as to permit a more accurate definition and quantification of calcifications. Differently than ultrasound, light propagates well beyond the calcification and enables the evaluation of not only the plain circumference of the calcium arch and its length but also the depth and thickness[29] to calculate the area[30] and the volume[24,31,32] of CAC. In some cases, the identification of calcium on the OCT image can be difficult, such as when the calcific deposit is deep and is covered by a lipid or necrotic nucleus (conditions that greatly attenuate the light signal) or in the case of non-homogeneous plaques (therefore with both fibro-lipidic and calcific components)[33]. Beyond these limitations, OCT is considered a more precise technique than IVUS in defining the calcific burden[24,34]. OCT-evaluable parameters have been shown to accurately predict response to balloon dilatation and stent expansion[35]. Fujino et al. validated a calcium score based on OCT, which considers circumference (> 180° = 2 points), thickness (> 0.5 mm = 1 point), and longitudinal length
The position of the imaging catheter (IVUS or OCT) with respect to an eccentric calcified plaque may also help in predicting the impact of the rotational atherectomy burr on the plaque. For example, if the imaging catheter is in contact with the wall opposite to that in which the greater calcific component is present, with a larger caliber burr than initially foreseen, it will be possible to obtain greater contact between the device itself and the calcific plaque, and therefore greater efficacy in calcium ablation[36]. Finally, intravascular imaging plays a fundamental role in the evaluation of the result of stenting and OCT has a much greater sensitivity than IVUS in detecting stent malapposition or under-expansion and in appreciating the effects of post-dilatation[35,37,38].
TECHNICAL CONSIDERATIONS FOR PERCUTANEOUS CORONARY INTERVENTION (PCI) OF HEAVILY CALCIFIC CORONARY LESIONS
Vascular access, guiding catheters, and guidewires
PCI of heavily calcified lesions requires careful planning. Femoral access may be considered in situations which may require a lumen guide catheter greater than 6 French (F, 1F = 0.3 mm), especially in the presence of small-caliber radial arteries or in case of extremely tortuous coronaries, in which may be necessary increased support afforded by the femoral approach. However, in current practice, PCI for calcified lesions is performed indifferently by both radial and femoral arterial access, with the obvious advantages associated with radial access in terms of risk of complications[39,40]. Extremely thin-walled radial sheaths are now available to allow a 7F guide catheter to be held in the inner lumen while having an outer diameter equal to that of a 6F sheath (GlideSheath Slender, Terumo Corp., Tokyo, Japan). Alternatively, a sheathless guiding catheter may be used, which does not require a percutaneous introducer, and which has a 7.5F internal working lumen with a smaller outer diameter than a 6F radial introducer (EauCath, ASAHI Intecc, Aichi, Japan). For rotational atherectomy with a burr larger than 1.5 mm or when the coronary anatomy is particularly complex, it is always good practice to use a guide catheter with a lumen greater than 6F, but a 1.75 burr can be used with 6F guide catheters without important limitations. For guidewire selection, it is preferable to use non-hydrophilic guides (e.g., Hi-Torque Balance Middleweight, Abbott Vascular, Santa Clara, CA, USA or Sion Blue, ASAHI Intecc or Runthrough NS, Terumo Corp.), as they provide better tactile feedback, thereby reducing the risk of sub-intimal passage of the guidewire or coronary dissection. If these guidewires are ineffective in crossing the calcified lesion, hydrophilic guidewires such as Sion (ASAHI Intecc) and Runtrough NS Hypercoat (Terumo Corp.) or polymeric guidewires such as Pilot 50 (Abbott Vascular) or Fielder FC can be used (ASAHI Intecc). The use of a balloon catheter or a micro-catheter can provide greater support and control of the guidewire and facilitate passage through the calcified lesion, especially if it is functionally occlusive.
Lesion preparation
Severely calcified lesions must be accurately prepared for stent implantation. In most cases, the difficulty in advancing the devices across the calcified lesion and the high risk of stent under-expansion require extensive plaque modification. Thus, it is intuitive that calcified lesions cannot be treated with direct stent implantation. The techniques available for the treatment of calcifications can be classified into two groups
Devices used for plaque modification of calcified lesions
“Balloon-based” techniques | Ablative techniques |
Cutting balloon • Wolverine, Boston Scientific | Rotational Atherectomy • Rotablator and ROTAPRO, Boston Scientific |
Scoring balloon • AngioSculpt, Spectranetics • Scoreflex, OrbusNeich | Orbital Atherectomy • Diamondback 360° Coronary Orbital Atherectomy System, Cardiovascular Systems |
Very high-pressure non-compliant balloon • OPN, SIS Medical | LASER Atherectomy • CVX-300 ELCA System, Spectranetics |
Lithotripsy balloon • Rx Shockwave, Shockwave Medical |
Balloon-based techniques
Theoretically, the first choice for the predilatation of calcified lesions should be a simple non-compliant balloon, especially in cases where an ablative technique could be unsafe, such as in patients with low left ventricular ejection fraction (LVEF), with a single patent vessel, or with acute coronary syndrome and a high thrombotic burden in which distal embolization can have deleterious consequences. Patients with macroscopic thrombus or dissections visible on angiography are also at greater risk of procedural complications with atherectomy techniques. Despite the slightly higher profile compared to conventional semi-compliant balloons, non-compliant balloons are preferred because they are characterized by a more uniform expansion even at high pressures. This is particularly important in calcific lesions where the expansion of a semi-compliant balloon at elevated pressures can lead to preferential expansion towards the point of least resistance of the plaque[44], where the calcific component is absent, thereby increasing the risk of dissection or vessel rupture. There are some specific balloons for extremely fibrotic or calcified lesions that exert a focal radial expansive force. This translates into even more controlled balloon expansion, overall, with a good efficacy and safety profile, thus reducing barotrauma and the risk of coronary dissections and perforations. The Cutting Balloon (Wolverine, Boston Scientific, Natick, MA, USA) incorporates microsurgical blades arranged longitudinally on its surface and creates incisions in the plaque upon inflation, allowing for greater lumen gain at lower pressures and with less barotrauma, both in simpler lesions[45] and in calcified ones[46]. IVUS analysis demonstrated that lesion modification with cutting balloons is associated with a greater reduction of plaque burden and greater lumen gain in calcified lesions compared with simple balloon angioplasty[47]. Theoretically, scoring balloons (AngioSulpt, Spectranetics, Colorado Springs, CO, USA, Scoreflex, OrbusNeich, Hong Kong, China) have a better navigability profile. They have nitinol metal wires on the surface of the balloon, which facilitate the anchoring of the closed device to the plaque and determine, upon inflation, incisions in the plaque itself and limit the risk of dissection or perforation[48]. Furthermore, there are non-compliant very high-pressure balloons (OPN, SIS Medical, Frauenfeld, Switzerland), made up of a double-layer structure that allows a rated burst pressure (RBP) of 35 atmospheres. This feature represents a unique option for the effective dilatation of under-expanded stents[49]. Data from a recent multicentre observational register have then confirmed its efficacy and ease of use for the preparation of severely calcified lesions, maintaining an excellent safety profile[50].
Rotational atherectomy
Historical perspective
Rotational atherectomy was developed in the pre-stent era as a tool to improve the outcomes of percutaneous procedures for dilating calcified atherosclerotic lesions. In 1988, Fourrier et al. reported the first series of RA in humans as a stand-alone therapy or supplemented by balloon dilations[51]. With the advent of the stent era and the awareness that the residual plaque burden at the time of stent implantation was directly proportional to the degree of neointimal proliferation, the cause of intrastent restenosis, it was hypothesized that maximum mechanical debulking could reduce the risk of restenosis, increasing the minimum luminal diameter (MLD) obtained at the end of the procedure and limiting the degree of barotrauma to the vessel[52]. Observational data confirmed the efficacy and safety of a pre-PTCA RA strategy for heavily calcified lesions, with success rates ≥ 90% and a low incidence of procedural complications and out-of-hospital Major Adverse Cardiac Events (MACE)[53]. Despite these procedural premises, studies did not demonstrate a lower rate of long-time mortality or target lesion revascularization (TLR) with the use of mechanical debulking compared to conventional balloon intervention[54], thus slowing down the diffusion of this technique at the beginning of the new millennium. The introduction of the first-generation DES led to a reduction in intrastent restenosis rates below 10%[55]. As a result, interventional cardiologists began to confidently treat more and more complex lesions such as tortuous and calcific vessels percutaneously, thus showing a renewed interest in atherectomy techniques, revisited, however, from a more modern perspective in order to obtain an optimal preparation of the vessel rather than and extensive plaque debulking. In the current era of 2nd and 3rd generation DES, RA is used as a technique for modifying heavy CAC to increase the number of lesions suitable for PCI and optimizing procedural results rather than as a technique aimed at preventing restenosis[56], in a strategy known as "Rota-stent". In the first decade of the 2000s, RA confirmed excellent results in terms of procedural success[57], even if this hardly translated into a consistent long-term benefit in terms of restenosis and MACE[58-60]. Indeed, the 2018 ESC/EACTS (European Society of Cardiology/European Association for Cardio-Thoracic Surgery) guidelines on myocardial revascularization only mention, without providing a clear recommendation, the use of RA in selected lesions, in particular markedly calcific ones, in order to adequately dilate the lesions before stent implantation[61]. In patients undergoing PCI, RA is currently used in less than 5% of cases[62], excluding some high-volume centers in North America where the prevalence of use exceeds 10%[63].
Technical fundamentals of Rotational Atherectomy
The Rotablator system (Boston Scientific Corporation, Natick, Boston, MA, USA) is the most widely used atherectomy device in current interventional practice for the treatment of calcified atherosclerotic lesions in the coronary arteries. It consists of an elliptical-shaped diamond-coated metal burr that rotates at very high speed and, advancing into the coronary lumen, acts as an abrasive surface against the calcified plaque. The burr is available in diameters ranging from 1.25 to 2.5 mm and is mounted on a transmission shaft (advancer) connected to a system that converts compressed gas into rotational energy. The burr size should be chosen according to a burr-to-artery ratio of 0.5-0.6. Commonly, a single 1.5 mm burr represents the right compromise to obtain sufficient plaque modification; however, a step-up approach starting with a
Randomized evidence supporting Rotational Atherectomy
In a landmark randomized study of 240 patients with moderate to severe calcifications (Rotational Atherectomy Prior to TAXUS Stent Treatment for Complex Native Coronary Artery Disease, ROTAXUS), lesion preparation with RA resulted in greater procedural success than with predilatation with a standard balloon (92.5% vs. 83.3%, P = 0.03), even if in terms of long-term results, both at 9 months and at 2 years, the clinical effect was not significantly better and the incidence of MACE was in general quite relevant regardless of the preparation strategy[59,67]. A comparison of a planned RA strategy vs. a bailout RA strategy for severely calcified non-crossable or non-dilatable lesions demonstrated less procedural time, less use of contrast agent and less incidence of coronary dissections requiring additional stenting[68]. More recently, the PREPARE-CALC study randomized 200 patients with severely calcified lesions to RA vs. angioplasty with cutting/scoring balloon. The results demonstrate that RA is superior in terms of procedural success compared to the cutting/scoring balloon strategy (98% vs. 81%) with shorter fluoroscopy times, but both strategies guarantee an excellent result at 9 months in terms of angiographic late loss and new incidence of revascularizations[69]. These data were confirmed at 2-year follow-up[70] and underscored the importance of the combination of operators’ experience, intravascular imaging guidance and excellent DES performance to obtain optimal long-term results in treating patients with severely calcified lesions. In summary, RA demonstrated a higher procedural success rate in randomized trials, confirmed by the incidence of a crossover rate to RA between 12% and 16% in the control arm.
Rotational Atherectomy procedure
The RA procedure is performed after administration of a standard dose of heparin (70-100 U/Kg), obtaining an activated clotting time (ACT) ≥ 300 s. Recommendations for an optimal RA procedure include[62,63]:
• A guide catheter with high support stably positioned in the coronary ostium, for example, the Extra Backup for the left coronary artery and the Amplatz Left for the right coronary artery;
• Stable positioning of the Rotawire in the distal segment of the vessel to be treated; the technical difficulties in manipulating the Rotawire can be overcome by wiring the vessel with a conventional 0.014” guidewire supported by an over-the-wire system (micro-catheter or balloon), which allows the conventional guide to be exchanged with the Rotawire;
• One or two burrs of increasing diameter, while maintaining a burr/artery ratio ≤ 0.5-0.6, as larger diameter burrs are more likely to cause complications[71];
• Burr rotation speed between 140,000 and 180,000 rpm;
• Gradual advancement of the burr using a pecking technique, i.e., a rapid staking movement of the burr of the Rotablator against the calcified lesion, avoiding continuous contact, which should result in the absence of decelerations > 5,000 rpm which are indeed associated with a greater risk of entrapment (stall) of the burr. The pecking maneuver should be repeated until the complete crossing of the calcific lesion is obtained without significant decelerations. In fact, this technique prevents the burr from being pushed in a continuous forward movement and, therefore, the possibility of crossing the entire lesion during the initial passage. In any case, short advancements of the active burr (≤ 15-30 s) are recommended, with a sufficient pause before the next burr activation, to allow burr cooling, clearance of the particles from the microcirculation, and sufficient time to check patient ECG and hemodynamic parameters. Finally, a “cleaning” or “polishing” run is always recommended in which the burr should freely move forward across the lesion without any deceleration.
Rotational Atherectomy contraindications
“Classical” contraindications to RA include lesions in the last remaining patent vessel, lesions in patients with depressed left ventricular function, lesions on venous bypass grafts, lesions with angiographic evidence of thrombus or significant dissection. Despite these contraindications, several case reports in the literature and the experience of expert operators can confirm the safety and efficacy of RA in exceptional circumstances such as heavy calcified non-dilatable lesions in venous grafts, iatrogenic coronary dissections, acute myocardial infarction and left main lesions in patients deemed unsuitable for surgical revascularization[61]. Therefore, RA remains an invaluable technical aid in the percutaneous revascularization of high-risk patients, such as those with a single patent vessel, low LVEF and serious comorbidities that fall within the modern definition of Complex Higher-risk and Indicated Patients (CHIP) and are currently being successfully treated with appropriate hemodynamic circulatory support[72].
Prevention and management of complications of Rotational Atherectomy
RA procedures are associated with the same spectrum of clinical complications as traditional PCI; however, coronary dissection and perforation, acute vessel occlusion and slow-flow/no-reflow may be more frequent as the risk of these complications is greater in case of severely calcified lesions[73] regardless of the use of RA[74]. In highly tortuous and angulated vessels, the risk of coronary perforation with RA is higher. A specific complication of RA is burr entrapment.
Slow-flow/no-reflow can determine a deterioration of patient hemodynamics as they compromise the contractility of the myocardial territory subtended by the treated vessel. The pathogenesis is multifactorial and includes not only distal embolization of pulverized plaque, but also coronary spasm and platelet activation and aggregation elicited by contact of the burr with the vessel wall. No-reflow can be treated with intracoronary vasodilators (nitroglycerin, adenosine, verapamil, nitroprusside), but the hypotension induced by these drugs can accentuate the slow-flow and, sometimes, the aid of mechanical circulatory support (MCS) may be necessary. Abciximab reduces the transient hypoperfusion observed during RA and periprocedural myocardial infarction[75], but a word of caution is needed when considering the use of glycoprotein IIb/IIIa inhibitors (GPI) during RA procedures because of their bleeding potential. Burr entrapment is a complication feared by operators but which can be effectively prevented with the application of meticulous technique[62,63] and which occurs more frequently in cases of aggressive advancement of the burr through eccentric lesions in tortuous, angulated and extremely calcified vessels. If the burr passes an incompletely ablated lesion, its retrieval proximal to the lesion may be impeded by the absence of the diamond surface on its posterior half, which makes retrograde ablation impossible. During its advancement through the calcified lesions, the burr must continue to rotate without decelerations
Orbital atherectomy
The Diamondback 360° Coronary Orbital Atherectomy (OA) System (Cardiovascular Systems Inc., St. Paul, MN, USA) is a percutaneous device currently indicated to facilitate the placement and implantation of coronary stents. The OA system uses a single-size (1.25 mm) diamond orbiting eccentric crown that runs eccentrically on a dedicated guidewire (ViperWire, Cardiovascular Systems Inc.); the system is compatible with 6 Fr guide catheters and is operated by an electric console; a special knob allows to control the forward and backward movements of the crown. The system involves a washing solution (ViperSlide, Cardiovascular Systems Inc.) which reduces friction while the orbiting crown ablates calcium; the system can operate at low or high speed (80,000 or 120,000 rpm, respectively). The mechanism of action of the orbiting crown is bi-directional differential sanding, which uses centrifugal force to ablate the hard calcified tissue and deflects the normal tissue, leaving it intact. The high speed creates a larger sanding diameter by increasing lateral pressure, while the slower manual advance of the orbital crown increases the radius of the orbit, eventually creating fractures of calcium[77]. Coronary OA can be assimilated to RA but has several potentially advantageous technical aspects that distinguish it. The orbiting crown allows a bidirectional ablation of the calcium, both in forward and backward motions, to reduce the risk of device entrapment. In addition, the orbiting eccentric crown creates pulsatile forces that affect deeper calcium and contributes to change in vessel compliance[78]. Thanks to the small dimensions of the crown, the blood flow is maintained during the calcium ablation, and this reduces thermal injury and allows the continuous washout of the micro-particles produced by ablation (which, however, are smaller than 2 microns, therefore smaller than those produced by RA), and therefore a lower risk of no-reflow. The specificity of orbital atherectomy is the variable lumen size that can be obtained with the single 1.25 mm crown according to the duration of ablation, the number of passes, and the rotational speed. Since centrifugal force is a function of both the speed of rotation and the device mass, faster speeds result in increased centrifugal force and ultimately a larger orbit. As a result, a larger lumen can be created by the same crown simply by rotating it at higher speeds. The OA system is currently used in the USA and Japan and has been clinically introduced in Europe in the last couple of years. Data on OA clinical outcomes are derived from the single-arm ORBIT I, first-in-man studies of 50 patients in India[79] and ORBIT II, 443 patients in the USA[80], and from a retrospective real-world registry of 458 patients, which confirmed the safety and efficacy of OA with low rates of angiographic complications and MACE[81]. Long-term results report a 3-year target lesion repeat revascularization (TLR) incidence of 7.8% and MACE of 23.5% in the ORBIT II study[82]. The ECLIPSE study[83], the largest ever randomized study in patients with severely calcified coronary lesions (clinicaltrials.gov: NCT03108456) has recently completed the enrollment of approximately 2,000 patients receiving OA or conventional angioplasty to prepare coronary arteries for stent implantation. Studies with OCT have shown that OA results in more profound tissue modification than RA and a lower incidence of malapposed stent struts[43]. Basically, this theoretically better vessel preparation could be associated with clinically superior long-term results; however, no randomized study has ever compared OA with RA. Post-marketing data from the USA report a not negligible incidence of complications, including both vascular (perforations related to excessive straightening of the ViperWire and dissections) and general events (arrhythmias, and death). The most reported failure modes included detachment and/or structural damage of the device components and device entrapment[84]. On this background, high speed is now generally avoided in tortuous lesions, severe angulations, and vessels smaller than 3.0 mm in diameter[84]. Not differently than with RA, attention to meticulous procedural technique is the key to effective safe utilization of OA.
Excimer coronary LASER Atherectomy
Technical fundamentals of ELCA and preliminary studies
LASER is the acronym for “Light Amplification by Stimulated Emission of Radiation”. Laser energy is produced when an active medium, excited by electrical energy, emits monochromatic coherent light. The only approved system for coronary application is the excimer laser (CVX-300 ELCA System, Spectranetics Inc., Colorado Springs, Colorado). Its laser unit uses XeCl (monochloride of Xenon) as the active medium at a wavelength of 308 nm and generates a pulsed energy emission of up to 80 mJ/mm2 with a maximum repetition rate of 40 Hz and width of pulse of 125-200 ns. This energy is called fluence. The system has a
The few randomized studies available, conducted over twenty years ago, have not demonstrated the superiority of revascularization with ELCA compared to conventional PTCA of complex lesions. In the multicenter AMRO study of 308 patients with stable angina, there was no difference in procedural success (80% with ELCA vs. 79%) or net lumen gain on angiography (0.40 mm vs. 0.48 mm), moreover with a transient occlusion rate 10 times higher (7% vs. 0.7%). At 6 months, there was a trend towards a higher incidence of restenosis with laser (51.6 vs. 41.3%)[91]. The ERBAC study compared PTCA with ELCA (as well as rotational atherectomy) in 685 patients with stable angina[92]. Procedural success rates were comparable (77% and 80%) between the ELCA and PTCA groups, as were in-hospital complication rates (4.3% and 3.1%). At 6 months, the target vessel revascularization rate was significantly higher in the ELCA group (46.0% vs. 31.9%)[92]. In a meta-analysis of 16 trials and 9,222 patients treated with various percutaneous interventional techniques, a significantly higher probability (OR 1.55, 95%CI: 1.09-2.20) of developing restenosis with ELCA compared to conventional PTCA was also documented[54].
Current indications to ELCA
The results of the randomized studies and of the meta-analysis do not support the extensive use of the ELCA, and since this is also a very expensive technology, its application remains limited to specific targeted indications. Among them, a common indication for ELCA is the presence of calcific lesions that cannot be crossed or dilated[93,94], chronic total occlusions (CTO) that cannot be crossed with a micro-catheter, intrastent restenosis and under-expanded stents[89], lesions with a high thrombotic burden in acute myocardial infarction in addition to or as an alternative to conventional thrombectomy devices[95,96]. The ELCA technique indeed permits the vaporization of thrombotic material, inhibits platelet aggregation, and ablates the underlying atherosclerotic plaque[97]. ELCA is now rarely used as a first-line strategy for highly calcified lesions but remains the only option when the lesion cannot be crossed -even with a micro-catheter- to allow placement of a RA guidewire. In a small study of 58 uncrossable/undilatable lesions with conventional balloons, very satisfactory results were obtained with ELCA, with even better efficacy if the use of the laser was followed by RA[98].
Chronic total occlusion (CTO) may typically be treated with the ELCA. Even after the passage of the guidewire through a CTO, the angioplasty procedure cannot sometimes be ultimately performed because of the impossibility of crossing the lesion with either a micro-catheter or a balloon[99]. ELCA is a valuable option in such circumstances because, differently than with RA, the laser catheter can be used over standard guidewires; it creates a channel through the CTO and allows other devices to ultimately cross the lesion[100].
ELCA can be used for in-stent restenosis due to neointimal hyperplasia[101] and in calcified non-expandable in-stent restenosis[102], especially when the latter is a consequence of stent under-expansion at time of original implantation[89]. A recent OCT study showed the superiority of ELCA over high pressure POBA in the treatment of non-dilatable intrastent restenosis and confirmed the action of ELCA in breaking the calcium behind the stent struts, especially during the administration of contrast agent, which allows for generating larger bubbles with a higher photomechanical effect[103,104].
Intra-vascular lithotripsy
General principles
Intra-Vascular Lithotripsy (IVL) is the most recent technique that has been developed to treat calcific atherosclerotic lesions. IVL operation mode is based on pulsatile mechanical energy delivered by miniaturized emitters positioned along a rapid exchange semi-compliant balloon (Rx Shockwave, Shockwave Medical, Santa Clara, California) and transmitted to the vessel by means of the inflated balloon inside the vessel. These emitters produce circumferential sound pressure waves that traverse the soft tissue and fracture the calcium of the tunica intima and especially of the tunica media within the vessel wall. Microfractures in calcium increase vessel compliance, allowing adequate dilatation of calcific lesions or passage of larger devices. Barotrauma on the vessel walls is minimized by the low inflation pressure of the semi-compliant balloon (4-6 atmospheres). Such a preferential effect on deep calcium is the great advantage of lithotripsy over plaque ablation techniques. Its use was first described in the treatment of calcified lesions of peripheral arteries[105] and subsequently extended to the treatment of calcified coronary artery disease.
Technical and procedural considerations
The coronary IVL system consists of a disposable catheter, connector cable, and pulse generator. The catheter does not differ in concept from a conventional balloon catheter, even though it has a larger profile and much more stiffness. The size of the IVL balloon is chosen according to the reference vessel diameter is chosen, with a ratio ≥ 1 being considered appropriate[106]. Once the lesion has been reached, the 12 mm long IVL catheter balloon is inflated to 4 atmospheres to allow contact with the vessel wall and optimization of sonic pulse wave energy delivery. Treatment cycles include one pulse per second for 10 s. After each cycle, the balloon is brought to 6 atmospheres. This helps to compress the fractured calcium before a new treatment cycle. A maximum of 8 treatment cycles (80 pulses) can be delivered for a single IVL catheter. For lesions longer than 12 mm, the catheter must therefore be repositioned to treat the lesion along its entire length. In the event that the IVL catheter is unable to initially cross a calcified lesion, it is possible to perform predilatation with low-profile angioplasty balloons or even a rotational atherectomy, which will facilitate positioning of the IVL catheter[107] (Rota-Shock technique). However, coronary IVL remains a balloon-based technique and therefore, a relatively simple technique with a short learning curve. These considerations, combined with the accruing efficacy and safety evidence, place IVL in the foreground among the techniques for treating calcified coronary lesions.
Clinical evidence
The feasibility of the IVL technique in patients with severely calcified coronary artery lesions was assessed in four single-arm prospective trials[108], which demonstrated the high procedural success and safety of the technique, with a reasonably predictable incidence of adverse events. DISRUPT CAD I is the first-in-man multicenter study on the use of coronary IVL before stent implantation in 60 patients with markedly calcified lesions ≤ 32 mm in length[109]. Clinical success (< 50% residual stenosis with no major in-hospital events) was 95% due to the presence of 3 post-procedural non-Q myocardial infarctions. It is important to note that no serious safety issues or technical complications such as coronary perforations, major dissections, and slow flow/no-reflow or additional MACE events, including target vessel revascularizations, were reported at 30 days. In the OCT sub-study, IVL was found to result in fractures in the calcific component of the plaque in 43% of cases, with multiple circumferential fractures in > 25% of cases. In particular, the effectiveness of the technique has been shown to be proportional to the calcium load, with a higher rate of fractures in cases with a higher degree of calcification. The acute gain of luminal area after the use of the IVL catheter was 2.1 mmq[110]. DISRUPT CAD II is the prospective study following the commercialization of the coronary IVL system in Europe. The study enrolled 120 patients with severe calcific coronary artery disease treated with IVL and stent implantation. In this case, the primary outcome was a composite of in-hospital MACE events (cardiac death, myocardial infarction, and target lesion revascularization). The procedural success, in terms of effective treatment of the calcific lesion with IVL, was 100%, and the fractures in the calcium were confirmed with OCT in 79% of cases. In all cases, the intra-stent residual stenosis was less than 30%. No perforations or slow flow/no-reflow phenomena occurred in any case and the incidence of MACE was 5.8% for the occurrence of 7 non-Q-wave myocardial infarction[111]. DISRUPT-CAD III was designed for regulatory approval of IVL and enrolled 431 patients from the United States, the United Kingdom, France, and Germany. The primary endpoints of freedom from MACE at 30 days (92.2%) for safety and procedural success for effectiveness (92.4%), respectively, were both met. The mean calcified segment length (~50 mm), thickness (~1 mm), and angle (~300°) were consistent with a high amount of CAC. OCT demonstrated calcium fractures after IVL in 67.4% of lesions and minimal stent area was independent of demonstrable fractures on OCT[112]. DISRUPT-CAD IV[113] was designed for regulatory approval of coronary IVL in Japan with a similar design and comparable results to DISRUPT-CAD III.
ALGORITHM FOR TREATMENT OF SEVERELY CALCIFIC CORONARY LESIONS
Any operator should plan the strategy of treatment of a severely calcified coronary lesion after having evaluated, as much accurately as possible, width of the circumference arc, longitudinal extension, depth, and thickness of calcification in the coronary wall. All these observations can only be obtained using an imaging technique, IVUS or OCT, to be chosen based on the operator’s experience and availability[114].
The impossibility of obtaining accurate imaging of the lesion because of the inability of the IVUS catheter to cross the lesion or to obtain optimal blood clearance for OCT image acquisition should promptly direct the operator towards an ablative technique such as atherectomy, or laser, if available. In the former case, aiming to streamline the procedure, the guidewire that has crossed the lesion must be exchanged with the dedicated guide of the atherectomy system, either RA or OA: this maneuver is greatly simplified by using a micro-catheter. If even lesion crossing with the micro-catheter is not possible, the alternatives are either the direct lesion wiring with the dedicated guidewire (Rotawire or Viperwire) of the atherectomy system (this is not a straightforward maneuver, especially with the Rotawire because of the difficulties in manipulating it through complex anatomies), or the use of the ELCA catheter on the guidewire which the crossing of the lesion was obtained with.
As an alternative to primary atherectomy, an attempt at predilatation with an undersized balloon, possibly a non-compliant one, can be considered to obtain a minimal luminal gain so as to be able to interrogate the vessel with the imaging catheter. The failure of this initial predilatation should promptly lead to an atherectomy technique [Figure 3].
Figure 3. Algorithm for treatment of severely calcific coronary lesions. The role of intravascular imaging and different and complementary plaque modification techniques is shown.
Once the morphological information on the calcification has been obtained, the use of non-compliant balloons or special balloons (cutting/scoring balloons) can be considered as an alternative to atherectomy when the arc of calcification is < 180° or when, although being the arc > 180°, the thickness of the calcification is < 0.5 mm and the length is < 5 mm and basically the operator is dealing with a small amount of calcification[27,29]. In the presence of a calcification arc > 180°, the key element is the topography of the calcium in the vessel wall, i.e., whether it is predominantly an intimal or a medial CAC. In the latter case, IVL can be considered the first choice due to its efficacy in addressing deep CAC, especially if luminal stenosis is not tight. In the former case of intimal CAC, it is preferable to use an atherectomy device as there is a substantial risk of IVL balloon damage once it encounters the irregular profile of the calcified intimal wall not yet effectively prepared. Nodular calcifications should lead to atherectomy techniques in the first instance[115].
Once the desired preparation technique has been performed (special balloon, atherectomy, IVL, or a combination thereof), the actual modification of the calcified plaque should be confirmed with an imaging technique, for example, by trying to demonstrate fractures in the CAC with OCT or a clear increase in vessel lumen with IVUS [Figure 4]. The key maneuver, however, is the confirmation of the optimal expansion of a non-compliant balloon [Figure 5], of a 1:1 sizing with the target vessel (“balloon testing” or “lesion palpation”), ideally with two orthogonal views, preparatory to stent implantation; alternatively, the vessel preparation should be repeated and implemented with, for example, a larger atherectomy burr or a IVL balloon.
Figure 4. Percutaneous revascularization of the calcific lesion shown in Figure 2B. In (A), baseline angiography shows a significant stenosis in proximal LAD (arrow). In (B), IVUS interrogation shows a concentric ring calcification. In (C), calcium fractures are evident at IVUS after Intravascular Lithotripsy and high-pressure non-compliant balloon dilatation. In (D), vessel lumen enlargement and optimal stent apposition are evident at IVUS. In (E) final angiographic result is shown.
Figure 5. Percutaneous revascularization of the calcific lesion shown in Figure 1. In (A), baseline angiography is shown. After Rotational Atherectomy (B), incomplete expansion of a non-compliant balloon is evident at conventional angiography (C) and after using stent-enhancement features (D). After Intravascular Lithotripsy, optimal balloon expansion is confirmed along the entire calcified stenotic segment (E and F). Final result after stent optimization is shown with stent-enhancement features (G) and conventional angiography (H).
Once the stent has been implanted, it is necessary to check the result with the intravascular imaging catheter to verify the optimal expansion and apposition of the stent struts to the vessel wall[35]. Any stent optimization that may be necessary will be carried out with conventional non-compliant or very high-pressure balloons. The use of an IVL balloon inside a newly implanted stent in order to optimize stent apposition by acting on CAC remaining under the stent struts is a technically feasible procedure[116], even if it should currently be considered off-label since it is not known whether the sound pressure waves delivered against the metal structure and the polymer of the DES can cause any structural alterations[117].
CONCLUSIONS
Patients with calcific coronary artery disease represent a subset with a high risk of adverse outcomes, both intra-procedural and in the long-term. The need to guarantee a targeted and tailored treatment based on the coronary anatomy of any individual patient is a current priority of the interventional community. The efficacy of RA in improving procedural success for the treatment of calcified lesions has been widely demonstrated. The advent of new technologies -and especially of IVL-, the application of techniques and materials initially developed for as complex procedures as CTO, the increasing experience of contemporary operators and the introduction of new generation DES with excellent technical and structural properties, are further contributing to improving outcomes of current PCI for calcific lesions.
DECLARATIONS
Authors’ contributions
Made substantial contributions to the conception and design of the study and wrote the manuscript: Andò G, Alagna G, De Rosa S, Pelliccia F, Gragnano F, Piccolo R, Moscarella E, Fabris E, Montone RA, Muscoli S, Spaccarotella C, Niccoli G
Provided administrative and material support and critically reviewed the manuscript: Sinagra G, Indolfi C, Porto I, Perrone Filardi P, Calabrò P
Availability of data and materials
Not applicable.
Financial support and sponsorship
None.
Conflicts of interest
All authors declared that there are no conflicts of interest.
Ethical approval and consent to participate
Not applicable.
Consent for publication
Not applicable.
Copyright
© The Author(s) 2023.
REFERENCES
1. Grüntzig AR, Senning A, Siegenthaler WE. Nonoperative dilatation of coronary-artery stenosis: percutaneous transluminal coronary angioplasty. N Engl J Med 1979;301:61-8.
2. Barbato E, Shlofmitz E, Milkas A, Shlofmitz R, Azzalini L, Colombo A. State of the art: evolving concepts in the treatment of heavily calcified and undilatable coronary stenoses - from debulking to plaque modification, a 40-year-long journey. EuroIntervention 2017;13:696-705.
3. Budoff MJ, Shaw LJ, Liu ST, et al. Long-term prognosis associated with coronary calcification: observations from a registry of 25,253 patients. J Am Coll Cardiol 2007;49:1860-70.
4. Greenland P, LaBree L, Azen SP, Doherty TM, Detrano RC. Coronary artery calcium score combined with Framingham score for risk prediction in asymptomatic individuals. JAMA 2004;291:210-5.
5. Généreux P, Madhavan MV, Mintz GS, et al. Ischemic outcomes after coronary intervention of calcified vessels in acute coronary syndromes. Pooled analysis from the HORIZONS-AMI (harmonizing outcomes with revascularization and stents in acute myocardial infarction) and ACUITY (acute catheterization and urgent intervention triage strategy) TRIALS. J Am Coll Cardiol 2014;63:1845-54.
6. Bourantas CV, Zhang YJ, Garg S, et al. Prognostic implications of coronary calcification in patients with obstructive coronary artery disease treated by percutaneous coronary intervention: a patient-level pooled analysis of 7 contemporary stent trials. Heart 2014;100:1158-64.
7. Guedeney P, Claessen BE, Mehran R, et al. Coronary calcification and long-term outcomes according to drug-eluting stent generation. JACC Cardiovasc Interv 2020;13:1417-28.
8. Généreux P, Redfors B, Witzenbichler B, et al. Two-year outcomes after percutaneous coronary intervention of calcified lesions with drug-eluting stents. Int J Cardiol 2017;231:61-7.
9. Huisman J, van der Heijden LC, Kok MM, et al. Impact of severe lesion calcification on clinical outcome of patients with stable angina, treated with newer generation permanent polymer-coated drug-eluting stents: a patient-level pooled analysis from TWENTE and DUTCH PEERS (TWENTE II). Am Heart J 2016;175:121-9.
10. Kuriyama N, Kobayashi Y, Yamaguchi M, Shibata Y. Usefulness of rotational atherectomy in preventing polymer damage of everolimus-eluting stent in calcified coronary artery. JACC Cardiovasc Interv 2011;4:588-9.
11. Wiemer M, Butz T, Schmidt W, Schmitz KP, Horstkotte D, Langer C. Scanning electron microscopic analysis of different drug eluting stents after failed implantation: from nearly undamaged to major damaged polymers. Catheter Cardiovasc Interv 2010;75:905-11.
12. Brilakis ES, Best PJ, Elesber AA, et al. Incidence, retrieval methods, and outcomes of stent loss during percutaneous coronary intervention: a large single-center experience. Catheter Cardiovasc Interv 2005;66:333-40.
13. Shao JS, Cheng SL, Sadhu J, Towler DA. Inflammation and the osteogenic regulation of vascular calcification: a review and perspective. Hypertension 2010;55:579-92.
14. Goodman WG, London G, Amann K, et al. Vascular calcification in chronic kidney disease. Am J Kidney Dis 2004;43:572-9.
15. Mori H, Torii S, Kutyna M, Sakamoto A, Finn AV, Virmani R. Coronary artery calcification and its progression: what does it really mean? JACC Cardiovasc Imaging 2018;11:127-42.
16. Sage AP, Tintut Y, Demer LL. Regulatory mechanisms in vascular calcification. Nat Rev Cardiol 2010;7:528-36.
17. Guagliumi G, Pellegrini D, Maehara A, Mintz GS. All calcified nodules are made equal and require the same approach: pros and cons. EuroIntervention 2023;19:e110-2.
18. Nelson AJ, Raggi P, Wolf M, Gold AM, Chertow GM, Roe MT. Targeting vascular calcification in chronic kidney disease. JACC Basic Transl Sci 2020;5:398-412.
19. Agatston AS, Janowitz WR, Hildner FJ, Zusmer NR, Viamonte M Jr, Detrano R. Quantification of coronary artery calcium using ultrafast computed tomography. J Am Coll Cardiol 1990;15:827-32.
20. Greenland P, Blaha MJ, Budoff MJ, Erbel R, Watson KE. Coronary calcium score and cardiovascular risk. J Am Coll Cardiol 2018;72:434-47.
21. Yu M, Li Y, Li W, Lu Z, Wei M, Zhang J. Calcification remodeling index characterized by cardiac CT as a novel parameter to predict the use of rotational atherectomy for coronary intervention of lesions with moderate to severe calcification. Korean J Radiol 2017;18:753-62.
22. Mintz GS, Popma JJ, Pichard AD, et al. Patterns of calcification in coronary artery disease. a statistical analysis of intravascular ultrasound and coronary angiography in 1155 lesions. Circulation 1995;91:1959-65.
23. Madhavan MV, Tarigopula M, Mintz GS, Maehara A, Stone GW, Généreux P. Coronary artery calcification: pathogenesis and prognostic implications. J Am Coll Cardiol 2014;63:1703-14.
24. Wang X, Matsumura M, Mintz GS, et al. In vivo calcium detection by comparing optical coherence tomography, intravascular ultrasound, and angiography. JACC Cardiovasc Imaging 2017;10:869-79.
25. Friedrich GJ, Moes NY, Mühlberger VA, et al. Detection of intralesional calcium by intracoronary ultrasound depends on the histologic pattern. Am Heart J 1994;128:435-41.
26. Hoffmann R, Mintz GS, Popma JJ, et al. Treatment of calcified coronary lesions with Palmaz-Schatz stents: an intravascular ultrasound study. Eur Heart J 1998;19:1224-31.
27. Zhang M, Matsumura M, Usui E, et al. Intravascular ultrasound-derived calcium score to predict stent expansion in severely calcified lesions. Circ Cardiovasc Interv 2021;14:e010296.
28. Tearney GJ, Regar E, Akasaka T, et al. Consensus standards for acquisition, measurement, and reporting of intravascular optical coherence tomography studies: a report from the International Working Group for intravascular optical coherence tomography standardization and validation. J Am Coll Cardiol 2012;59:1058-72.
29. Fujino A, Mintz GS, Matsumura M, et al. A new optical coherence tomography-based calcium scoring system to predict stent underexpansion. EuroIntervention 2018;13:e2182-9.
30. Kobayashi Y, Okura H, Kume T, et al. Impact of target lesion coronary calcification on stent expansion. Circ J 2014;78:2209-14.
31. Yabushita H, Bouma BE, Houser SL, et al. Characterization of human atherosclerosis by optical coherence tomography. Circulation 2002;106:1640-5.
32. Krishnamoorthy P, Vengrenyuk Y, Ueda H, et al. Three-dimensional volumetric assessment of coronary artery calcification in patients with stable coronary artery disease by OCT. EuroIntervention 2017;13:312-9.
33. Sharma SK, Vengrenyuk Y, Kini AS. IVUS, OCT, and coronary artery calcification: is there a bone of contention? JACC Cardiovasc Imaging 2017;10:880-2.
34. Kume T, Okura H, Kawamoto T, et al. Assessment of the coronary calcification by optical coherence tomography. EuroIntervention 2011;6:768-72.
35. Räber L, Mintz GS, Koskinas KC, et al. Clinical use of intracoronary imaging. Part 1: guidance and optimization of coronary interventions. An expert consensus document of the European Association of Percutaneous Cardiovascular Interventions. EuroIntervention 2018;14:656-77.
36. Maria GL, Scarsini R, Banning AP. Management of calcific coronary artery lesions: is it time to change our interventional therapeutic approach? JACC Cardiovasc Interv 2019;12:1465-78.
37. Ali ZA, Maehara A, Généreux P, et al. Optical coherence tomography compared with intravascular ultrasound and with angiography to guide coronary stent implantation (ILUMIEN III: OPTIMIZE PCI): a randomised controlled trial. Lancet 2016;388:2618-28.
38. Bergmark B, Dallan LAP, Pereira GTR, et al. Decision-making during percutaneous coronary intervention guided by optical coherence tomography: insights from the LightLab initiative. Circ Cardiovasc Interv 2022;15:872-81.
39. Andò G, Cortese B, Frigoli E, et al. Acute kidney injury after percutaneous coronary intervention: Rationale of the AKI-MATRIX (acute kidney injury-minimizing adverse hemorrhagic events by TRansradial access site and systemic implementation of angioX) sub-study. Catheter Cardiovasc Interv 2015;86:950-7.
40. Andò G, Costa F, Trio O, Oreto G, Valgimigli M. Impact of vascular access on acute kidney injury after percutaneous coronary intervention. Cardiovasc Revasc Med 2016;17:333-8.
41. Okamoto N, Ueda H, Bhatheja S, et al. Procedural and one-year outcomes of patients treated with orbital and rotational atherectomy with mechanistic insights from optical coherence tomography. EuroIntervention 2019;14:1760-7.
42. Sotomi Y, Cavalcante R, Shlofmitz RA, et al. Quantification by optical coherence tomography imaging of the ablation volume obtained with the Orbital Atherectomy System in calcified coronary lesions. EuroIntervention 2016;12:1126-34.
43. Kini AS, Vengrenyuk Y, Pena J, et al. Optical coherence tomography assessment of the mechanistic effects of rotational and orbital atherectomy in severely calcified coronary lesions. Catheter Cardiovasc Interv 2015;86:1024-32.
44. Fitzgerald PJ, Ports TA, Yock PG. Contribution of localized calcium deposits to dissection after angioplasty. An observational study using intravascular ultrasound. Circulation 1992;86:64-70.
45. Mauri L, Bonan R, Weiner BH, et al. Cutting balloon angioplasty for the prevention of restenosis: results of the Cutting Balloon Global Randomized Trial. Am J Cardiol 2002;90:1079-83.
46. Karvouni E, Stankovic G, Albiero R, et al. Cutting balloon angioplasty for treatment of calcified coronary lesions. Catheter Cardiovasc Interv 2001;54:473-81.
47. Okura H, Hayase M, Shimodozono S, et al. Mechanisms of acute lumen gain following cutting balloon angioplasty in calcified and noncalcified lesions: an intravascular ultrasound study. Catheter Cardiovasc Interv 2002;57:429-36.
48. Fonseca A, de Ribamar Costa Jr J, Abizaid A, et al. Intravascular ultrasound assessment of the novel AngioSculpt scoring balloon catheter for the treatment of complex coronary lesions. J Invasive Cardiol 2008;20:21-7.
49. Secco GG, Ghione M, Mattesini A, et al. Very high-pressure dilatation for undilatable coronary lesions: indications and results with a new dedicated balloon. EuroIntervention 2016;12:359-65.
50. Secco GG, Buettner A, Parisi R, et al. Clinical experience with very high-pressure dilatation for resistant coronary lesions. Cardiovasc Revasc Med 2019;20:1083-7.
51. Fourrier JL, Bertrand ME, Auth DC, Lablanche JM, Gommeaux A, Brunetaud JM. Percutaneous coronary rotational angioplasty in humans: preliminary report. J Am Coll Cardiol 1989;14:1278-82.
52. Prati F, Di Mario C, Moussa I, et al. In-stent neointimal proliferation correlates with the amount of residual plaque burden outside the stent: an intravascular ultrasound study. Circulation 1999;99:1011-4.
53. MacIsaac AI, Bass TA, Buchbinder M, et al. High speed rotational atherectomy: outcome in calcified and noncalcified coronary artery lesions. J Am Coll Cardiol 1995;26:731-6.
54. Bittl JA, Chew DP, Topol EJ, Kong DF, Califf RM. Meta-analysis of randomized trials of percutaneous transluminal coronary angioplasty versus atherectomy, cutting balloon atherotomy, or laser angioplasty. J Am Coll Cardiol 2004;43:936-42.
55. Stone GW, Moses JW, Ellis SG, et al. Safety and efficacy of sirolimus- and paclitaxel-eluting coronary stents. N Engl J Med 2007;356:998-1008.
56. Kuntz RE, Gibson CM, Nobuyoshi M, Baim DS. Generalized model of restenosis after conventional balloon angioplasty, stenting and directional atherectomy. J Am Coll Cardiol 1993;21:15-25.
57. Iannaccone M, Barbero U, D’ascenzo F, et al. Rotational atherectomy in very long lesions: results for the ROTATE registry. Catheter Cardiovasc Interv 2016;88:E164-72.
58. Abdel-Wahab M, Baev R, Dieker P, et al. Long-term clinical outcome of rotational atherectomy followed by drug-eluting stent implantation in complex calcified coronary lesions. Catheter Cardiovasc Interv 2013;81:285-91.
59. Abdel-Wahab M, Richardt G, Joachim Büttner H, et al. High-speed rotational atherectomy before paclitaxel-eluting stent implantation in complex calcified coronary lesions: the randomized ROTAXUS (Rotational Atherectomy Prior to Taxus Stent Treatment for Complex Native Coronary Artery Disease) trial. JACC Cardiovasc Interv 2013;6:10-9.
60. Arora S, Panaich SS, Patel N, et al. Coronary atherectomy in the United States (from a nationwide inpatient sample). Am J Cardiol 2016;117:555-62.
61. Neumann FJ, Sousa-Uva M, Ahlsson A, et al. 2018 ESC/EACTS guidelines on myocardial revascularization. Eur Heart J 2019;40:87-165.
62. Barbato E, Carrié D, Dardas P, et al. European expert consensus on rotational atherectomy. EuroIntervention 2015;11:30-6.
63. Sharma SK, Tomey MI, Teirstein PS, et al. North American expert review of rotational atherectomy. Circ Cardiovasc Interv 2019;12:e007448.
64. Cavusoglu E, Kini AS, Marmur JD, Sharma SK. Current status of rotational atherectomy. Catheter Cardiovasc Interv 2004;62:485-98.
65. Kovach JA, Mintz GS, Pichard AD, et al. Sequential intravascular ultrasound characterization of the mechanisms of rotational atherectomy and adjunct balloon angioplasty. J Am Coll Cardiol 1993;22:1024-32.
66. Eltchaninoff H, Cribier A, Koning R, et al. Angioscopic evaluation of rotational atherectomy followed by additional balloon angioplasty versus balloon angioplasty alone in coronary artery disease: a prospective, randomized study. J Am Coll Cardiol 1997;30:888-93.
67. de Waha S, Allali A, Büttner HJ, et al. Rotational atherectomy before paclitaxel-eluting stent implantation in complex calcified coronary lesions: two-year clinical outcome of the randomized ROTAXUS trial. Catheter Cardiovasc Interv 2016;87:691-700.
68. Allali A, Abdel-Wahab M, Sulimov DS, et al. Comparison of bailout and planned rotational atherectomy for heavily calcified coronary lesions: a single-center experience. J Interv Cardiol 2017;30:124-33.
69. Abdel-Wahab M, Toelg R, Byrne RA, et al. High-speed rotational atherectomy versus modified balloons prior to drug-eluting stent implantation in severely calcified coronary lesions. Circ Cardiovasc Interv 2018;11:e007415.
70. Allali A, Richardt G, Toelg R, et al. High-speed rotational atherectomy versus modified balloons for plaque preparation of severely calcified coronary lesions: two-year outcomes of the randomised PREPARE-CALC trial. EuroIntervention 2023;18:e1365-7.
71. Safian RD, Feldman T, Muller DW, et al. Coronary angioplasty and Rotablator atherectomy trial (CARAT): immediate and late results of a prospective multicenter randomized trial. Catheter Cardiovasc Interv 2001;53:213-20.
72. O’Neill WW, Kleiman NS, Moses J, et al. A prospective, randomized clinical trial of hemodynamic support with Impella 2.5 versus intra-aortic balloon pump in patients undergoing high-risk percutaneous coronary intervention: the PROTECT II study. Circulation 2012;126:1717-27.
73. Sakakura K, Inohara T, Kohsaka S, et al. Incidence and determinants of complications in rotational atherectomy: insights from the national clinical data (J-PCI registry). Circ Cardiovasc Interv 2016;9:e004278.
74. Giannini F, Candilio L, Mitomo S, et al. A practical approach to the management of complications during percutaneous coronary intervention. JACC Cardiovasc Interv 2018;11:1797-810.
75. Koch KC, vom Dahl J, Kleinhans E, et al. Influence of a platelet GPIIb/IIIa receptor antagonist on myocardial hypoperfusion during rotational atherectomy as assessed by myocardial Tc-99m sestamibi scintigraphy. J Am Coll Cardiol 1999;33:998-1004.
76. Sulimov DS, Abdel-Wahab M, Toelg R, Kassner G, Geist V, Richardt G. Stuck rotablator: the nightmare of rotational atherectomy. EuroIntervention 2013;9:251-8.
77. Sotomi Y, Shlofmitz RA, Colombo A, Serruys PW, Onuma Y. Patient selection and procedural considerations for coronary orbital atherectomy system. Interv Cardiol 2016;11:33-8.
78. Shlofmitz E, Martinsen BJ, Lee M, et al. Orbital atherectomy for the treatment of severely calcified coronary lesions: evidence, technique, and best practices. Expert Rev Med Devices 2017;14:867-79.
79. Parikh K, Chandra P, Choksi N, Khanna P, Chambers J. Safety and feasibility of orbital atherectomy for the treatment of calcified coronary lesions: the ORBIT I trial. Catheter Cardiovasc Interv 2013;81:1134-9.
80. Chambers JW, Feldman RL, Himmelstein SI, et al. Pivotal trial to evaluate the safety and efficacy of the orbital atherectomy system in treating de novo, severely calcified coronary lesions (ORBIT II). JACC Cardiovasc Interv 2014;7:510-8.
81. Lee MS, Shlofmitz E, Kaplan B, Alexandru D, Meraj P, Shlofmitz R. Real-world multicenter registry of patients with severe coronary artery calcification undergoing orbital atherectomy. J Interv Cardiol 2016;29:357-62.
82. Lee M, Généreux P, Shlofmitz R, et al. Orbital atherectomy for treating de novo, severely calcified coronary lesions: 3-year results of the pivotal ORBIT II trial. Cardiovasc Revasc Med 2017;18:261-4.
83. Généreux P, Kirtane AJ, Kandzari DE, et al. Randomized evaluation of vessel preparation with orbital atherectomy prior to drug-eluting stent implantation in severely calcified coronary artery lesions: design and rationale of the ECLIPSE trial. Am Heart J 2022;249:1-11.
84. Khalid N, Javed H, Rogers T, et al. Adverse events with orbital atherectomy: an analytic review of the MAUDE database. EuroIntervention 2020;16:e325-7.
85. Rawlins J, Din JN, Talwar S, O’Kane P. Coronary intervention with the excimer laser: review of the technology and outcome data. Interv Cardiol 2016;11:27-32.
86. Grundfest WS, Litvack F, Forrester JS, et al. Laser ablation of human atherosclerotic plaque without adjacent tissue injury. J Am Coll Cardiol 1985;5:929-33.
87. Isner JM, Donaldson RF, Deckelbaum LI, et al. The excimer laser: gross, light microscopic and ultrastructural analysis of potential advantages for use in laser therapy of cardiovascular disease. J Am Coll Cardiol 1985;6:1102-9.
88. Goldberg SL, Colombo A, Akiyama T. Stent under-expansion refractory to balloon dilatation: a novel solution with excimer laser. J Invasive Cardiol 1998;10:269-73.
89. Latib A, Takagi K, Chizzola G, et al. Excimer laser LEsion modification to expand non-dilatable stents: the ELLEMENT registry. Cardiovasc Revasc Med 2014;15:8-12.
90. Mintz GS, Kovach JA, Javier SP, et al. Mechanisms of lumen enlargement after excimer laser coronary angioplasty. An intravascular ultrasound study. Circulation 1995;92:3408-14.
91. Appelman YEA, Piek JJ, David GK, et al. Randomised trial of excimer laser angioplasty versus balloon angioplasty for treatment of obstructive coronary artery disease. Lancet 1996;347:79-84.
92. Reifart N, Vandormael M, Krajcar M, et al. Randomized comparison of angioplasty of complex coronary lesions at a single center. Excimer laser, rotational atherectomy, and balloon angioplasty comparison (ERBAC) study. Circulation 1997;96:91-8.
93. Bilodeau L, Fretz EB, Taeymans Y, Koolen J, Taylor K, Hilton DJ. Novel use of a high-energy excimer laser catheter for calcified and complex coronary artery lesions. Catheter Cardiovasc Interv 2004;62:155-61.
94. Ahmed WH, al-Anazi MM, Bittl JA. Excimer laser-facilitated angioplasty for undilatable coronary narrowings. Am J Cardiol 1996;78:1045-6.
95. Topaz O, Ebersole D, Das T, et al. Excimer laser angioplasty in acute myocardial infarction (the CARMEL multicenter trial). Am J Cardiol 2004;93:694-701.
96. Dörr M, Vogelgesang D, Hummel A, et al. Excimer laser thrombus elimination for prevention of distal embolization and no-reflow in patients with acute ST elevation myocardial infarction: results from the randomized LaserAMI study. Int J Cardiol 2007;116:20-6.
97. Ambrosini V, Cioppa A, Salemme L, et al. Excimer laser in acute myocardial infarction: single centre experience on 66 patients. Int J Cardiol 2008;127:98-102.
98. Fernandez JP, Hobson AR, McKenzie D, et al. Beyond the balloon: excimer coronary laser atherectomy used alone or in combination with rotational atherectomy in the treatment of chronic total occlusions, non-crossable and non-expansible coronary lesions. EuroIntervention 2013;9:243-50.
99. Gruberg L, Mehran R, Dangas G, et al. Effect of plaque debulking and stenting on short- and long-term outcomes after revascularization of chronic total occlusions. J Am Coll Cardiol 2000;35:151-6.
100. Shen ZJ, García-García HM, Schultz C, van der Ent M, Serruys PW. Crossing of a calcified “balloon uncrossable” coronary chronic total occlusion facilitated by a laser catheter: a case report and review recent four years’ experience at the Thoraxcenter. Int J Cardiol 2010;145:251-4.
101. Radke PW, Kaiser A, Frost C, Sigwart U. Outcome after treatment of coronary in-stent restenosis; results from a systematic review using meta-analysis techniques. Eur Heart J 2003;24:266-73.
102. Mehran R, Dangas G, Mintz GS, et al. Treatment of in-stent restenosis with excimer laser coronary angioplasty versus rotational atherectomy: comparative mechanisms and results. Circulation 2000;101:2484-9.
103. Lee T, Shlofmitz RA, Song L, et al. The effectiveness of excimer laser angioplasty to treat coronary in-stent restenosis with peri-stent calcium as assessed by optical coherence tomography. EuroIntervention 2019;15:e279-88.
104. Veerasamy M, Gamal AS, Jabbar A, Ahmed JM, Egred M. Excimer laser with and without contrast for the management of under-expanded stents. J Invasive Cardiol 2017;29:364-9.
105. Brodmann M, Werner M, Brinton TJ, et al. Safety and performance of lithoplasty for treatment of calcified peripheral artery lesions. J Am Coll Cardiol 2017;70:908-10.
106. Kereiakes DJ, Virmani R, Hokama JY, et al. Principles of intravascular lithotripsy for calcific plaque modification. JACC Cardiovasc Interv 2021;14:1275-92.
107. Chen G, Zrenner B, Pyxaras SA. Combined rotational atherectomy and intravascular lithotripsy for the treatment of severely calcified in-stent neoatherosclerosis: a mini-review. Cardiovasc Revasc Med 2019;20:819-21.
108. Kereiakes DJ, Di Mario C, Riley RF, et al. Intravascular lithotripsy for treatment of calcified coronary lesions: patient-level pooled analysis of the disrupt CAD studies. JACC Cardiovasc Interv 2021;14:1337-48.
109. Brinton TJ, Ali ZA, Hill JM, et al. Feasibility of shockwave coronary intravascular lithotripsy for the treatment of calcified coronary stenoses. Circulation 2019;139:834-6.
110. Ali ZA, Brinton TJ, Hill JM, et al. Optical coherence tomography characterization of coronary lithoplasty for treatment of calcified lesions: first description. JACC Cardiovasc Imaging 2017;10:897-906.
111. Ali ZA, Nef H, Escaned J, et al. TCT-27 safety and effectiveness of coronary intravascular lithotripsy for treatment of severely calcified coronary stenoses: the disrupt CAD II study. J Am Coll Cardiol 2019;74:B27.
112. Hill JM, Kereiakes DJ, Shlofmitz RA, et al. Intravascular lithotripsy for treatment of severely calcified coronary artery disease. J Am Coll Cardiol 2020;76:2635-46.
113. Saito S, Yamazaki S, Takahashi A, et al. Intravascular lithotripsy for vessel preparation in severely calcified coronary arteries prior to stent placement- primary outcomes from the Japanese disrupt CAD IV study. Circ J 2021;85:826-33.
114. Shlofmitz E, Ali ZA, Maehara A, Mintz GS, Shlofmitz R, Jeremias A. Intravascular imaging-guided percutaneous coronary intervention: a universal approach for optimization of stent implantation. Circ Cardiovasc Interv 2020;13:e008686.
115. Lee T, Mintz GS, Matsumura M, et al. Prevalence, predictors, and clinical presentation of a calcified nodule as assessed by optical coherence tomography. JACC Cardiovasc Imaging 2017;10:883-91.
116. Forero MN, Wilschut J, Van Mieghem NM, Daemen J. Coronary lithoplasty: a novel treatment for stent underexpansion. Eur Heart J 2019;40:221.
Cite This Article
How to Cite
Download Citation
Export Citation File:
Type of Import
Tips on Downloading Citation
Citation Manager File Format
Type of Import
Direct Import: When the Direct Import option is selected (the default state), a dialogue box will give you the option to Save or Open the downloaded citation data. Choosing Open will either launch your citation manager or give you a choice of applications with which to use the metadata. The Save option saves the file locally for later use.
Indirect Import: When the Indirect Import option is selected, the metadata is displayed and may be copied and pasted as needed.
About This Article
Special Issue
Copyright
Data & Comments
Data
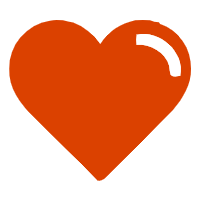
Comments
Comments must be written in English. Spam, offensive content, impersonation, and private information will not be permitted. If any comment is reported and identified as inappropriate content by OAE staff, the comment will be removed without notice. If you have any queries or need any help, please contact us at [email protected].