Crack the rock: the role of coronary lithotripsy for heavy calcified lesions
Abstract
Since the first balloon angioplasty 40 years ago, percutaneous coronary interventions (PCI) applicability continues to expand, even in the context of complex coronary anatomies, previously considered unsuitable for PCI. A very challenging scenario for PCI is the treatment of heavily calcified coronary stenosis that is associated with early complications and late adverse events. Countless tools and techniques have been introduced to treat calcified coronary stenosis, and among these, a new and promising technique obtained from lithotripsy technology employed for the treatment of uretero-renal calculi has been introduced: the intravascular lithotripsy (IVL). IVL utilizes acoustic shockwaves in a balloon-based delivery system that induces calcium fractures, facilitating stent advancement and expansion. This review aims to describe the device used for IVL, the results of clinical studies published, and the possible clinical use.
Keywords
INTRODUCTION
Percutaneous coronary intervention (PCI), first introduced in 1977 by Andreas Grüntzig[1], has substantially evolved over the past 40 years and its applicability continues to expand, even in the context of some complex coronary anatomies, previously considered unsuitable for PCI[2]. With an aging society, more and more frequently, interventional cardiologists are facing complex coronary lesions that include bifurcations, left main stenosis, thrombotic lesions, chronic total occlusions, and heavily calcified plaques[3]. These kinds of lesions represent a tough challenge for operators in terms of procedural success, complications, and long-term risk of restenosis[4]. Recently, the feasibility and success of these complex PCI have been essentially improved by the introduction of advanced techniques, approaches and adjunctive specific devices, particularly for the treatment of severely calcified lesions.
CALCIUM: ELEMENT OF RISK FOR PROCEDURAL SUCCESS
A particular challenge faced by interventional cardiologists is the treatment of calcified lesions: moderate to severe coronary artery calcifications (CAC) are documented in more than 30% of patients who underwent coronary angiography and a severe calcification has been observed in up to 15% of procedures[5]. CAC increase exponentially among elderly people, especially those affected by diabetes mellitus and advanced renal disease[6], and the presence of CAC is an independent predictor of adverse prognosis even after multivariate Cox regression analysis[7]. The presence of CAC hampers wires and device crossing, limits stent apposition and expansion and alters drug delivery and elution by delamination of drug-eluting polymers on drug-eluting stents[8], increasing early complications (perforations, dissections) and late adverse events (late thrombosis, restenosis) compared with noncalcified lesions[9,10].
Calcium quantification and distribution is a fundamental point of procedural planning[11], but coronary angiography has some limitations in visualizing coronary calcium that is better detected by means of invasive Intravascular imaging techniques such as IntraVascular UltraSound (IVUS) and Optical Coherence Tomography (OCT)[12,13]. These techniques provide adjunctive data on distribution and thickness of the calcified plaque.
CURRENTLY AVAILABLE TECHNOLOGIES TO MODIFY CALCIFIED PLAQUE
In severely calcified lesions, plain balloon angioplasty is seldom effective for lesion dilatation because the lesion could be resistant to high-pressure dilatation (in case of circumferential calcification) or because the balloon expansion is asymmetric towards the compliant vessel opposite the arc of calcium (in case of eccentric calcification). To address this clinical need, different tools and devices have been tested: high-pressure non-compliant (NC) balloon dilation, super high-pressure (OPN) balloon, cutting or scoring balloons, atherectomy techniques (rotational, orbital), laser and intravascular lithotripsy (IVL) balloons. All these devices have pros and cons, so the choice requires a careful lesion analysis and an individualized planning of the interventional strategy.
NC, cutting, scoring and OPN balloons increase the rate of procedural success compared with compliant balloons, but often, the force to disrupt calcium is insufficient to achieve vessel expansion. Besides their still limited efficacy, the rate of peri-procedural complications such as dissection or perforation, is not negligible[14].
More effective tools are debulking devices such as rotational and orbital atherectomy that allow to alter plaque morphology, creating fractures in the calcified lesion and a path through uncrossable lesions by other devices, increasing the probability of complete stent expansion with a high procedural success[8]. Particularly hard lesions can increase the risk of failure and/or incarceration of the burr (especially for rotational atherectomy, which does not have the possibility of bidirectional advancement). Ideal lesions for atherectomy are therefore serrated plaques with superficial calcification. Currently, their use is limited by the need for a learning curve and by the rate of coronary complications that include myocardial infarction, slow flow, flow-limiting dissection, distal embolization, and perforation. Furthermore, a randomised clinical trial performed in patients with heavily calcified coronary stenosis failed to demonstrate the superiority of rotational atherectomy versus conventional balloon dilatation before stent implantation[15] and randomized data for orbital atherectomy versus standard of care are lacking. Two other drawbacks of this method are the need for a dedicated wire and the inability to protect side branches with a second wire.
A new and promising device for the treatment of highly calcified coronary lesions is the intravascular lithotripsy (IVL) balloon, which features high efficacy, low complication rates, and simplicity of its use.
INTRAVASCULAR LITHOTRIPSY BALLOON: DEVICE DESCRIPTION
IVL is a technique derived from extracorporeal shock wave lithotripsy used for calcium modification that delivers acoustic pressure waves (shock waves) aimed to disrupt the calcium by creating multiplane micro-fractures in the plaque[16]. The first extracorporeal shock wave lithotripsy was reported in the 1980s for the treatment of urolithiasis using high-energy acoustic shockwaves[17]. The extracorporeal lithotripters generate a waveform characterized by a brief pulse lasting about 5-10 μs and characterized by a near-instantaneous jump to a peak positive pressure (compressive wave) with an abrupt transition (the “shock”), consisting of a falling pressure to zero in ≈1 μs, followed by a negative pressure trough (“tensile wave”) in almost 3 μs[18]. Shockwaves travel safely through soft tissue with negligible effect due to similar acoustic impedance between water and soft tissues, whereas the fracturing effect appears when sonic waves hit tissues with different acoustic impedance, such as the calcified tissue.
The application of lithotripsy to endovascular medicine refers to the use of a balloon-based technique characterized by miniaturized electrohydraulic lithotripters integrated onto the shaft of a balloon angioplasty catheter system. The balloon is filled with contrast and saline in order to allow apposition to the vessel wall and provide a similar acoustic fluid-tissue interface to deliver the sonic pressure waves created by the miniaturized lithotripters. The system could generate peak positive pressure in the range of 50 atm[19].
The IVL system (Shockwave Medical, Santa Clara, CA, USA) is made up of three components: a generator, a connector cable, and a single-use sterile disposable catheter that embeds the lithotripsy emitters[20]. The semi-compliant balloon catheter, which is 6 Fr compatible and can be used over a 0.014" guidewire, incorporates two radiopaque lithotripsy emitters 6 mm apart and two standard markers placed proximally and distally to the edge of the balloon. Compared to non-compliant balloon crossing profiles
The IVL procedure is a quite simple procedure that does not require high-level additional training for interventional cardiologists but just some simple explanations. The first step is the choice of the shockwave balloon that has to be sized according to the reference vessel diameter (ratio 1:1). Then, the IVL catheter is introduced into the target vessel over a 0.014-inch guidewire and is correctly placed across the target stenosis using balloon marker bands as visual guides. As the acoustic shockwaves are propagated through fluid and are impaired by air, the following step, essential to endure optimal transmission of the sonic wave, is to clear the air out of the catheter. Then, the balloon can be inflated up to 4 atm to obtain the correct apposition to the vessel wall. By pushing a button on the connector cable, the lithotripsy emitters are activated, and after a cycle of 10 pulses, the balloon can be inflated up to 6 atm (reference pressure) in order to increase balloon compliance and to check the symmetrical expansion that confirms calcium modification. A minimum of two IVL cycles are recommended to treat the target stenosis (max 80 pulses). At the end, the balloon must be deflated carefully to allow the exit of small air bubbles[21] [Figure 1].
Figure 1. Shockwave in a de-novo undilatable lesion. A heavily calcified lesion on the left anterior descending (A) has been treated by multiple pre-dilatation using non-compliant balloons (2.0 mm × 15 mm and 2.25 mm × 15 mm, NC Quantum Apex, inflated up to 20 atmospheres) without effective lesion dilatation and a “dog bone” effect (B). Five cycles of 10 pulses of intravascular lithotripsy with a 2.5 mm × 12 mm expanded up to 6 atmospheres (C) obtained a successful dilatation of the lesion. The procedure has been completed with 2 drug eluting stents (2.25 mm × 32 mm and 2.5 mm × 12 mm, Synergy, Boston) with a good final result (D).
HOW SHOCK WAVE CREATE CALCIUM FRACTURE
Different mechanisms, generally inter-related, are involved in the fragmentation of the calcific plaques by acoustic shockwaves: squeezing, cavitation, fatigue, and spallation[18]. Squeezing depends on the different speeds of sonic wave propagation between calcific deposits and soft tissue: shock waves move faster across calcium, causing a circumferential force on the calcified tissue and shear stress evolving in an axial splitting failure[22]. Cavitation refers to the generation of bubbles in the fluid, produced by the negative pressure of the acoustic wave. The collapse of these bubbles generates a micro-jet of fluid that strikes the calcium surface with a velocity upward of 100 m/s and a secondary shock wave, with an amplitude comparable with the original one[23]. Differently, fatigue is a process of progressive development of cracks located in correspondence of little imperfections of the solid calcific deposit. With multiple shock waves, the micro-fractures evolve into macro-fractures, creating cracks large enough to induce failure. This is why repeated shock waves are necessary to progressively fragment solid calcific deposits and the technique uses multiple stress cycles[24]. Finally, spallation is an effect of the reflection of the shock wave from the rear of the calcific deposit, generating a large negative tensile stress[22]. All these effects impact superficial and deep calcifications, allowing better stent expansion and luminal gaining, representing a significant advantage over atherectomy devices that target only superficial calcium. Other advantages of the shock wave are: the possibility of protecting side branch with second wire, no requirement for a specialized wire, and reduced risk of distal embolization since calcium remains within the vessel after shock disruption.
The method also has some limitations, the main of which is that if the IVL device cannot pass through a severely calcified lesion, extensive vessel preparation may be required: pre-dilatation is performed in
CLINICAL STUDIES
Feasibility and safety of the IVL system have been demonstrated in the four DISRUPT-CAD trials that led to the European approval of the device for the treatment of heavy calcified coronary lesions [13,25-27]. Globally, these trials were multicenter prospective single-arm studies that enrolled patients with de-novo calcified lesions (lesion length < 40 mm with vessel diameter between 2.5 and 4.0 mm) [Table 1]. An important inclusion criterion was the circumferential distribution of calcium fluoroscopically detected on both sides of the arterial wall or by the presence of ≥ 270 degrees of calcium on intravascular imaging. In general, the procedural success was very high, ranging from 92% to 95%, with a low rate of complications. On average, 1.3 ± 0.6 catheters were used with a mean number of pulses of 75 ± 43. Intravascular imaging studies confirmed the effectiveness of the device showing intraplaque calcium fracture, in particular in the most heavily calcified lesions[26]Figure 2. An interesting observation was that the fracture was detected by intravascular imaging in less than 70% of lesions but without differences in final angiographic result or clinical outcome compared to patients in whom there was not a macroscopic modification of the calcified lesions[13]. This suggests that the absence of imaging fracture detection is not a sign of ineffective therapy because the sonic waves may produce microfractures that are beyond the resolution power of imaging devices.
Figure 2. Shockwave for the treatment of an unexpanded coronary stent. A heavily calcified lesion on the circumflex artery (A) has been treated by multiple pre-dilatation using non-compliant balloons (2.5 mm × 15 mm and 3 mm × 15 mm, NC Quantum Apex, inflated up to 16 atmospheres). Then a drug eluting stent (B) was implanted (3.5 mm × 24 mm, Synergy Boston, expanded up to 20 atmospheres) without satisfactory expansion (C and D). Despite multiple post-dilatation using non-compliant balloons (4.0 mm × 12 mm and
Characteristics of the DISRUPT CAD studies
Disrupt I | Disrupt II | Disrupt III | Disrupt IV | |
Year of publishing | 2019 | 2019 | 2020 | 2021 |
N sites | 7 | 15 | 47 | 8 |
Patients | 60 | 120 | 384 | 64 |
Age (years) | 72 (66-79)* | 72 ± 10 | 71 ± 9 | 75 ± 8 |
Male sex | 80 (48) | 78 (94) | 77 (294) | 75 (48) |
Hyperlipidaemia | 80 (48) | 72 (86) | 89 (342) | 86 (55) |
Hypertension | 80 (48) | 80 (96) | 89 (342) | 83 (53) |
Diabetes mellitus | 30 (18) | 32 (38) | 40 (154) | 48 (31) |
Renal insufficiency | 10 (6) | 8 (10) | 26 (101) | 23 (15) |
Lesion length | 18 (14-25)* | 20 ± 10 | 26 ± 12 | 28 ± 10 |
Diameter stenosis | 73 (59-77)* | 60 ± 12 | 65 ± 11 | 66 ± 11 |
Procedural success | 95 (57) | 113 (94) | 92 (354) | 94 (60) |
Follow-up duration (days) | 180 | 30 | 30 | 30 |
MACE at follow-up | 2 (5) | 8 (4) | 8 (30) | 6 (4) |
TVR | 0 | 1 (1) | 2 (6) | 0 |
Angiographic complications | 0 | 2 (2) | 1 (2) | 0 |
Many exclusion criteria (acute myocardial infarction within 30 days, renal failure, left ventricular ejection fraction < 40%, unprotected left main, and chronic total occlusion) limit the applicability of the results of DISRUPT-CAD studies.
Data from real-world populations came from different studies, most of which were multicentre, both prospective[28-32] and retrospective[33-36], enrolling all consecutive IVL PCI, often without clinical or angiographic exclusion criteria [Table 2]. Other minor studies have analysed the efficacy and safety of IVL in special populations, reported later in the text. The retrospective design of some studies and the small number of patients enrolled in the prospective ones with a single-arm protocol sometimes limit their value. Globally, the reported procedural success and major adverse cardiovascular events (MACE) are similar compared to the DISRUPT CAD studies, confirming the feasibility of the IVL even in the context of more complex patients and procedures.
Clinical characteristics of patients enrolled in multicentre studies
Author | Aksoy | Aziz | Iwanczyk | Cubero-Gallego | Rola | El Jattari | Basavar-Ajaiah | Honton | Tian |
Year | 2019 | 2020 | 2021 | 2021 | 2021 | 2022 | 2022 | 2022 | 2023 |
Study type | Pros | Retr | Retr | Pros | Retr | Pros | Retr | Pros | Pros |
Sites | 3 | 6 | 3 | 5 | 2 | 5 | 8 | 11 | 3 |
Patients | 71 | 190 | 46 | 109 | 52 | 134 | 273 | 202 | 20 |
Lesions | 78 | 200 | 46 | 109 | 54 | 134 | 273 | 202 | 20 |
Age (years) | 76 ± 10 | 72 ± 10 | 71.1 ± 7.4 | 79 ± 1 | 71 ± 3 | 74 ± 9 | 72 ± 9 | 74 ± 9 | 65 ± 8 |
Male sex | 72 (51) | 72 (137) | 65 (30) | 83 (90) | 67 (35) | 76 (102) | 79 (216) | 77 (155) | 80 (16) |
Hyperlipidaemia | 63 (45) | NA | 94 (43) | 79.82 (87) | 96 (52) | NA | NA | 64 (129) | 45 (9) |
Hypertension | 93 (66) | 92 (172) | 96 (44) | 88.99 (97) | 90 (47) | NA | 78 (214) | 81 (163) | 70 (14) |
Diabetes | 34 (24) | 50 (95) | 54 (25) | 58 (63) | 56 (29) | 34 (45) | 40 (110) | 35 (71) | 50 (10) |
CKD | 35 (25) | 16 (30) | 24 (11) | 32.11 (35) | 19 (10) | NA | 17 (45) | 25 (50) | 0 |
Lesion length | 21 ± 16 | NA | NA | 26 ± 9 | NA | NA | 32 ± 11 | 22 ± 14 | 27 ± 7 |
% Stenosis | 72 ± 13 | NA | 80 (70-90)* | 60 | 83 ± 10 | NA | NA | 81 ± 11 | 73 ± 12 |
LM | 16.7 (13) | 15 (28) | 12 (25) | 11.72 (15) | 35 (18) | 6.7 (9) | 17 (47) | 11 (25) | 0 |
CTO | 3 (2) | 7 (14) | 7 (3) | 3 (4) | 10 (5) | NA | 4 (12) | NA | 0 |
ISR | 22 (17) | 23 (46) | 17 (6) | NA | NA | 30 (40) | 21 (58) | 23 (50) | 0 |
ACS | 31 (22) | 48 (91) | 82.6 (38) | 60.56 (66) | 83 (43) | 33.7 (45) | 48 (132) | 21 (42) | 0 |
STEMI | 1 (1) | NA | 0 | 11 (12) | 6 (3) | 4 (5) | 11 (39) | NA | 0 |
UA/NSTEMI | 29.6 (21) | NA | 78 (36) | 50 (54) | 77 (40) | 29.9 (40) | 37 (103) | NA | 0 |
Success | 78 (61) | 99 (189) | 89 (45) | 99 (108) | 98 (51) | 88 (118) | 99 (270) | 96 (194) | 95 (19) |
MACE | 1 (1) | 3 (5) | 2 (1) | 6 (7) | 6 (3) | 3 (4) | 11 (30) | 15 (31) | 5 (1) |
Complications | 5 (4) | 3 (6) | 4 (2) | 2 (3) | 4 (2) | 2 (2) | 3 (8) | 6 (12) | 0 |
FU (days) | 30 | 222 | 30 | 600 | 30 | 30 | 687 | 365 | 180 |
Additional randomized clinical trials (Clinical Trials.gov ID NCT04960319, NCT04253171, NCT04428177) and multicentre observational registries (NCT05016726, NCT05828186, NCT04698902) are currently underway that may provide more robust data on the efficacy of IVL PCI in the real world and its advantages compared with other debulking methods.
It is surprising that a cost-effectiveness analysis is lacking in most of the trials conducted by now. A good cost-effectiveness analysis should not include just the cost of the device but also the cost of any eventual re-intervention necessary for target lesion failure after S-IVL or the cost of other complementary techniques for treating severe calcification. Furthermore, the cost of any complications, duration of hospitalization, and impact on mortality should be taken into account. Comparing the costs of single devices, the cheapest are NC balloons, followed by cutting and scoring balloons, which cost 20 times more. The cost of atherectomy devices is higher than balloons but cheaper than IVL devices. Anyway, evaluating the cost of the whole IVL-PCI, we agree with Kassimis et al. that lithotripsy device may be more cost-effective than other treatments of severe calcified stenosis as the need for additional devices is reduced, the rate of complications is lower, procedural success is higher and long-term clinical results are improved. Specific analyses are needed to confirm this hypothesis[37].
SPECIAL POPULATIONS
The feasibility and safety of IVL has also been tested in different specific conditions as:
- Left main stenosis. A potential advantage of the IVL over other plaque modification techniques is the treatment of large vessels as left main. Only few studies have specifically been conducted on IVL use on left main calcified lesions[38-40], but in almost all the studies, the PCI success rate was 100% with no or very few complications rate despite the complexity of the lesions treated. A possible concern for the use of IVL on LM lesions is the prolonged vessel occlusion needed to deliver the required energy that could lead to severe ischaemia. In this case, the application of abbreviated IVL cycles could be used to avoid significant ischemia.
- Acute myocardial infarction. Although acute coronary syndrome presentation was an exclusion criterion in the approval studies, the use of IVL balloons in these kinds of lesions reserves promising potential. In the real-world multicentre registries, the percentage of acute coronary syndromes was around 50%, but the percentage of ST-elevation myocardial infarction was significantly lower. One of the larger multicentre retrospective analyses[41] was performed in the UK on 72 STEMI patients in whom IVL was used for CAC modification of the culprit lesion during primary PCI. The rate of no-reflow was low (4%), with no cases of coronary perforation and one case of stent thrombosis. The rate of 30-day MACE was quite high (18%), mainly due to a high rate of mortality (17%). Anyway, this finding may be explained by the older age and the higher rate of comorbidity of this cohort of patients.
- Stent under-expansion. Stent under-expansion is a worrisome condition during PCI associated with in-stent restenosis and mostly stent thrombosis[42]. In this setting, no specific technique or device is effective to adequately dilate the under-expanded stent and no consensus exists about the optimal approach to the treatment of such lesions. In this field, IVL represents a promising resource, with different studies showing the effectiveness of IVL in the correct treatment of these lesions [Figure 3], even though the procedural success is lower than for de-novo lesions[28,43-45]. Among these studies, the CRUNCH registry[46] is the largest, enrolling 70 patients who received IVL therapy to treat stent under-expansion. The results showed a device success of 92%, with significant minimum lumen diameter and stent expansion increase without in-hospital IVL-related procedural complications or MACE.
Figure 3. OCT image of severe calcific stenosis before and after IVL balloon expansion. (A) OCT image of severe calcific stenosis with an almost circumferential distribution over 260° (as also indicated by the arcs on the circumference). (B) Post IVL OCT image, showing intracoronary calcium fragmentation.
- Chronic total occlusion. Moderate to severe calcifications and undilatable lesions are frequently found in chronic total occlusions (CTO), and often increase the difficulty of PCI. IVL is increasingly used in CTO PCI and some studies have shown encouraging outcomes[47,48]. Kostantinis et al. analysed 3,301 CTO PCI procedures performed at 14 Centers between the years 2020 and 2022[49]. IVL was used in 82 procedures (2.5%), particularly in 10% of all heavily calcified lesions and 11% of all balloon undilatable lesions. Procedural success was 90%, with only 2% of Ellis Class 2 perforations, whereas the in-hospital MACE rate was 3.7%, with one death. In a case report, IVL has also been utilized in a CTO-PCI as a last resort to externally crush in the sub-intimal space a heavy calcified previously implanted stent[50].
COMPLICATIONS
The IVL device has a favourable safety profile and the rate of procedural complications is very low. The most frequent angiographic complications associated with the use of the device are the occurrence of coronary perforations or dissections, but their rate is in the range of 1% in the majority of studies and in particular in the DISRUPT CAD trials [Tables 1 and 2]. No cases of slow flow have been observed in the DISRUPT CAD series of studies (12,25-27), probably because the large calcium fragments generated by IVL remain in situ compared to microparticles generated by atherectomy devices that could embolize distally.
The use of IVL might be associated with atrial or ventricular capture in patients with bradycardia, as initially described by Wilson et al. and defined as “shocktopics”[51]. In the DISRUPT CAD III Trial, an IVL-induced capture was observed in 41% of cases[13], but no sustained ventricular arrhythmias were observed. In patients with implantable defibrillators or pace-makers, this asynchronous capture might interfere with the sensing capabilities of the device even though no electrical current leaves the IVL catheter. The probability of sustained ventricular arrhythmias induction is extremely rare, but a single case of ventricular fibrillation has been correlated to the use of IVL due to ventricular ectopy on a T wave[52].
CONCLUSIONS
IVL is a new technology aimed at treating heavily calcified coronary stenosis. The device is highly effective, easy to use (without a learning curve), and associated with a favourable safety profile. The growing experience with the device enables its application across a range of different clinical scenarios, particularly for the treatment of under-expanded coronary stent that nowadays has no further treatment options.
DECLARATIONS
Authors’ contributionsSubstantially contributed to the conception of the manuscript, drafted and critically reviewed: Sciahbasi A
Read and approved the final version of the manuscript: Sciahbasi A, Minardi S
Availability of data and materialsNot applicable.
Financial support and sponsorshipNone.
Conflicts of interestBoth authors declared that there are no conflicts of interest.
Ethical approval and consent to participateNot applicable.
Consent for publicationNot applicable.
Copyright© The Author(s) 2023.
REFERENCES
1. Grüntzig AR, Senning Å, Siegenthaler WE. Nonoperative dilatation of coronary-artery stenosis: percutaneous transluminal coronary angioplasty. N Engl J Med 1979;301:61-8.
2. Seung KB, Park DW, Kim YH, et al. Stents versus coronary-artery bypass grafting for left main coronary artery disease. N Engl J Med 2008;358:1781-92.
3. Baber U, Kini AS, Sharma SK. Stenting of complex lesions: an overview. Nat Rev Cardiol 2010;7:485-96.
4. Wilensky RL, Selzer F, Johnston J, et al. Relation of percutaneous coronary intervention of complex lesions to clinical outcomes (from the NHLBI Dynamic Registry). Am J Cardiol 2002;90:216-21.
5. Généreux P, Madhavan MV, Mintz GS, et al. Ischemic outcomes after coronary intervention of calcified vessels in acute coronary syndromes. Pooled analysis from the HORIZONS-AMI (Harmonizing Outcomes with Revascularization and Stents in Acute Myocardial Infarction) and ACUITY (Acute Catheterization and Urgent Intervention Triage Strategy) TRIALS. J Am Coll Cardiol 2014;63:1845-54.
6. Allison MA, Criqui MH, Wright CM. Patterns and risk factors for systemic calcified atherosclerosis. Arterioscler Thromb Vasc Biol 2004;24:331-6.
7. Bourantas CV, Zhang YJ, Garg S, et al. Prognostic implications of coronary calcification in patients with obstructive coronary artery disease treated by percutaneous coronary intervention: a patient-level pooled analysis of 7 contemporary stent trials. Heart 2014;100:1158-64.
8. Barbato E, Shlofmitz E, Milkas A, Shlofmitz R, Azzalini L, Colombo A. State of the art: evolving concepts in the treatment of heavily calcified and undilatable coronary stenoses - from debulking to plaque modification, a 40-year-long journey. EuroIntervention 2017;13:696-705.
9. Madhavan MV, Tarigopula M, Mintz GS, Maehara A, Stone GW, Généreux P. Coronary artery calcification: pathogenesis and prognostic implications. J Am Coll Cardiol 2014;63:1703-14.
10. Guedeney P, Claessen BE, Mehran R, et al. Coronary calcification and long-term outcomes according to drug-eluting stent generation. JACC Cardiovasc Interv 2020;13:1417-28.
11. Barbato E, Gallinoro E, Abdel-Wahab M, et al. Management strategies for heavily calcified coronary stenoses: an EAPCI clinical consensus statement in collaboration with the EURO4C-PCR group. Eur Heart J ;2023:ehad342.
12. Ali ZA, Brinton TJ, Hill JM, et al. Optical coherence tomography characterization of coronary lithoplasty for treatment of calcified lesions: first description. JACC Cardiovasc Imaging 2017;10:897-906.
13. Hill JM, Kereiakes DJ, Shlofmitz RA, et al. Intravascular lithotripsy for treatment of severely calcified coronary artery disease. J Am Coll Cardiol 2020;76:2635-46.
14. Rheude T, Rai H, Richardt G, et al. Super high-pressure balloon versus scoring balloon to prepare severely calcified coronary lesions: the ISAR-CALC randomised trial. EuroIntervention 2021;17:481-8.
15. Abdel-Wahab M, Richardt G, Joachim Büttner H, et al. High-speed rotational atherectomy before paclitaxel-eluting stent implantation in complex calcified coronary lesions: the randomized ROTAXUS (Rotational Atherectomy Prior to Taxus Stent Treatment for Complex Native Coronary Artery Disease) trial. JACC Cardiovasc Interv 2013;6:10-9.
16. Kereiakes DJ, Virmani R, Hokama JY, et al. Principles of intravascular lithotripsy for calcific plaque modification. JACC Cardiovasc Interv 2021;14:1275-92.
17. Powers CJ, Tinterow MM, Burpee JF. Extracorporeal shock wave lithotripsy: a study of renal stone differences. Kans Med 1989;90:19-22.
18. Karimi Galougahi K, Patel S, Shlofmitz RA, et al. Calcific plaque modification by acoustic shock waves: intravascular lithotripsy in coronary interventions. Circ Cardiovasc Interv 2021;14:e009354.
19. Dini CS, Tomberli B, Mattesini A, et al. Intravascular lithotripsy for calcific coronary and peripheral artery stenoses. EuroIntervention 2019;15:714-21.
21. Forero MNT, Daemen J. The coronary intravascular lithotripsy system. Interv Cardiol 2019;14:174-81.
22. Sapozhnikov OA, Maxwell AD, MacConaghy B, Bailey MR. A mechanistic analysis of stone fracture in lithotripsy. J Acoust Soc Am 2007;121:1190-202.
23. Chitnis PV, Cleveland RO. Quantitative measurements of acoustic emissions from cavitation at the surface of a stone in response to a lithotripter shock wave. J Acoust Soc Am 2006;119:1929-32.
24. Zohdi TI, Szeri AJ. Fatigue of kidney stones with heterogeneous microstructure subjected to shock-wave lithotripsy. J Biomed Mater Res B Appl Biomater 2005;75:351-8.
25. Brinton TJ, Ali ZA, Hill JM, et al. Feasibility of shockwave coronary intravascular lithotripsy for the treatment of calcified coronary stenoses. Circulation 2019;139:834-6.
26. Ali ZA, Nef H, Escaned J, et al. Safety and effectiveness of coronary intravascular lithotripsy for treatment of severely calcified coronary stenoses: the disrupt CAD II study. Circ Cardiovasc Interv 2019;12:e008434.
27. Saito S, Yamazaki S, Takahashi A, et al. Intravascular lithotripsy for vessel preparation in severely calcified coronary arteries prior to stent placement - primary outcomes from the japanese disrupt CAD IV study. Circ J 2021;85:826-33.
28. Aksoy A, Salazar C, Becher MU, et al. Intravascular lithotripsy in calcified coronary lesions: a prospective, observational, multicenter registry. Circ Cardiovasc Interv 2019;12:e008154.
29. Cubero-Gallego H, Calvo-Fernandez A, Tizon-Marcos H, et al. Real-world multicenter coronary lithotripsy registry: long-term clinical follow-up. J Invasive Cardiol 2022;34:E701-8.
30. El Jattari H, Holvoet W, De Roeck F, et al. Intracoronary lithotripsy in calcified coronary lesions: a multicenter observational study. J Invasive Cardiol 2022;34:E24-31.
31. Honton B, Lipiecki J, Monségu J, et al. Mid-term outcome of de novo lesions vs. in stent restenosis treated by intravascular lithotripsy procedures: insights from the French shock initiative. Int J Cardiol 2022;365:106-11.
32. Tian F, Zhou SS, Liu JH, et al. Treatment of severely calcified coronary artery disease by intravascular lithotripsy primary outcomes and 180-day follow-up from the Chinese SOLSTICE trial. J Geriatr Cardiol 2023;20:32-9.
33. Aziz A, Bhatia G, Pitt M, et al. Intravascular lithotripsy in calcified-coronary lesions: a real-world observational, European multicenter study. Catheter Cardiovasc Interv 2021;98:225-35.
34. Rola P, Włodarczak A, Kulczycki JJ, et al. Feasibility of the intravascular lithotripsy in coronary artery disease. Short-term outcomes of the Lower-Silesia Shockwave Registry. Kardiol Pol 2021;79:1133-5.
35. Iwańczyk S, Włodarczak A, Hiczkiewicz J, et al. Feasibility of intravascular lithotripsy for calcific coronary lesions: a multi-institutional experience. Catheter Cardiovasc Interv 2021;98:E540-7.
36. Basavarajaiah S, Ielasi A, Raja W, et al. Long-term outcomes following intravascular lithotripsy (IVL) for calcified coronary lesions: a real-world multicenter European study. Catheter Cardiovasc Interv 2022;101:250-60.
37. Kassimis G, Ziakas A, Didagelos M, et al. Shockwave coronary intravascular lithotripsy system for heavily calcified de novo lesions and the need for a cost-effectiveness analysis. Cardiovasc Revasc Med 2022;37:128-34.
38. Salazar CH, Gonzalo N, Aksoy A, et al. Feasibility, safety, and efficacy of intravascular lithotripsy in severely calcified left main coronary stenosis. JACC Cardiovasc Interv 2020;13:1727-9.
39. Cosgrove CS, Wilson SJ, Bogle R, et al. Intravascular lithotripsy for lesion preparation in patients with calcific distal left main disease. EuroIntervention 2020;16:76-9.
40. Rola P, Włodarczak A, Kulczycki JJ, et al. Efficacy and safety of shockwave intravascular lithotripsy (S-IVL) in calcified unprotected left main percutaneous coronary intervention - short-term outcomes. Postepy Kardiol Interwencyjnej 2021;17:344-8.
41. Cosgrove C, Hanratty CG, Hill JM, et al. Intravascular lithotripsy for treatment of calcific coronary lesions in ST elevation myocardial infarction. Catheter Cardiovasc Interv 2022;99:322-8.
42. Souteyrand G, Amabile N, Mangin L, et al. Mechanisms of stent thrombosis analysed by optical coherence tomography: insights from the national PESTO French registry. Eur Heart J 2016;37:1208-16.
43. Ielasi A, Moscarella E, Testa L, et al. IntravaScular lithotripsy for the management of UndILatable coronary StEnt: the SMILE registry. Cardiovasc Revasc Med 2020;21:1555-9.
44. Wańha W, Tomaniak M, Wańczura P, et al. Intravascular lithotripsy for the treatment of stent underexpansion: the multicenter IVL-DRAGON registry. J Clin Med 2022;11:1779.
45. Hinton J, Mariathas M, Chan E, et al. Novel application of intravascular lithotripsy in stent under-expansion: a single-center experience. Catheter Cardiovasc Interv 2022;101:243-9.
46. Tovar Forero MN, Sardella G, Salvi N, et al. Coronary lithotripsy for the treatment of underexpanded stents: the international & multicentre CRUNCH registry. EuroIntervention 2022;18:574-81.
47. Øksnes A, Cosgrove C, Walsh S, et al. Intravascular lithotripsy for calcium modification in chronic total occlusion percutaneous coronary intervention. J Interv Cardiol 2021;2021:9958035.
48. Rola P, Włodarczak A, Barycki M, et al. Shockwave intravascular lithotripsy as a novel strategy for balloon undilatable heavily calcified chronic total occlusion lesions. Cardiol J 2021.
49. Kostantinis S, Simsek B, Karacsonyi J, et al. Intravascular lithotripsy in chronic total occlusion percutaneous coronary intervention: insights from the PROGRESS-CTO registry. Catheter Cardiovasc Interv 2022;100:512-9.
50. Garbo R, Di Russo C, Sciahbasi A, Fedele S. The last resort during complex retrograde percutaneous coronary chronic total occlusion intervention: extraplaque intracoronary lithotripsy to externally crush a heavy calcified occluded stent. Catheter Cardiovasc Interv 2022;99:497-501.
51. Wilson SJ, Spratt JC, Hill J, et al. Incidence of "shocktopics" and asynchronous cardiac pacing in patients undergoing coronary intravascular lithotripsy. EuroIntervention 2020;15:1429-35.
Cite This Article
How to Cite
Download Citation
Export Citation File:
Type of Import
Tips on Downloading Citation
Citation Manager File Format
Type of Import
Direct Import: When the Direct Import option is selected (the default state), a dialogue box will give you the option to Save or Open the downloaded citation data. Choosing Open will either launch your citation manager or give you a choice of applications with which to use the metadata. The Save option saves the file locally for later use.
Indirect Import: When the Indirect Import option is selected, the metadata is displayed and may be copied and pasted as needed.
About This Article
Special Issue
Copyright
Data & Comments
Data
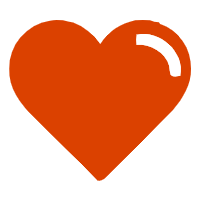
Comments
Comments must be written in English. Spam, offensive content, impersonation, and private information will not be permitted. If any comment is reported and identified as inappropriate content by OAE staff, the comment will be removed without notice. If you have any queries or need any help, please contact us at [email protected].