Role of advanced three-dimensional visualization modalities in congenital heart surgery
Abstract
The utility of three-dimensional visualization modalities such as three-dimensional printing, augmented reality, and virtual reality has transformed the field of surgery in the past few years. These modalities have demonstrated value in preoperative planning, procedural training, and surgical guidance in many surgical specialties. This review explores the current use of three-dimensional visualization modalities in surgery and further investigates its use and potential in the subspecialty of congenital heart defect surgery.
Keywords
INTRODUCTION
The evolution of three-dimensional (3D) visualization modalities has awarded surgeons the luxury of not needing to mentally reconstruct anatomy or memorize the location of pathology after studying a series of two-dimensional (2D) images[1,2]. This process can be mentally draining and ultimately affect other cognitive processes, which leads to an increase in complications and longer surgery times[1].
In medicine, 3D printing allows the production of surgical instruments, implants, and pathological organs using imaging files from computed tomography (CT) or magnetic resonance imaging (MRI). These solid and graspable objects give surgeons a better understanding of the anatomy and help improve patient-physician communication. Hence, it has the potential to reduce the duration of surgery while minimizing the risk of infection and complication[3,4].
The development of advanced visualization technology, which includes virtual reality (VR) and augmented reality (AR), has also revolutionized surgical training as it allows repeated use with varying difficulties and the ability to self-evaluate after every use[1,5]. VR refers to a computer-simulated experience in a synthetic world. In surgery, VR has been experimented for surgical planning, as well as in education and preoperative training[6,7]. It can also be used as a distraction tool for patients during procedures to minimize the need for anesthesia, resulting in faster recovery time and decreased anesthetic complications[8]. Postoperatively, it can be used to decrease pain and reduce psychological stress experienced primarily in cardiac surgery[9]. Like VR, AR involves virtual images but those superimposed in the real world instead[10]. Its use has increased in laparoscopic and minimally invasive procedures as it allows for precise identification of important internal structures that are often difficult to see on a 2D image. This translates to easier surgical planning, improved surgical outcomes, and better patient education[11-16].
This review provides an overview of the current applications of 3D printing, VR, and AR in surgery, and looks further into its role in congenital heart surgery.
3D printing in surgery
The use of 3D printing has gained widespread popularity in the field of congenital heart defect surgery due to its ability to display spatial relationships and complex cardiac anatomy[17]. 3D cardiac models have been used for presurgical planning of a variety of congenital heart defect, ranging from simple atrial septal defect or ventricular septal defect (VSD) lesions to complex cardiac lesions, with the most common application being for double outlet right ventricle (DORV) repair planning[18].
To create a 3D cardiac model, imaging using cardiac CT, MRI, or 3D echocardiogram is performed, given their ability to distinguish blood from the myocardium and vessel wall. Segmentation of the images is then performed, and the result is later converted from a Digital Imaging and Communication in Medicine (DICOM) file to a file format compatible with printing. The printable file is then uploaded to the software program of a 3D printer, and materials are selected based on the utility of the heart model[19].
In cardiology, surgeons use patient-specific 3D printed cardiac models as surgical guides to determine the pathology, areas to resect, and to gain hands-on practice before surgery[19]. Patients with congenital heart diseases often have a variety of complex cardiac anatomy that is difficult to visualize using 2D. This is especially crucial in cardiac defect repair surgery for children where thorax and heart sizes are small, making it difficult to visualize the actual surgical field[19].
Garekar et al.[20] discussed the unique cardiac anatomy of five patients with DORV and remote VSD. The creation of 3D cardiac models for these patients allowed surgeons to understand the relationship between the great arteries, ventricular mass, and VSD, decide on the surgical approach, and assist with presurgical planning. For patients who underwent surgery, intraoperative anatomy was in agreement with the model, and it helped boost the team’s confidence intraoperatively[20].
Hoashi et al.[21] found the greatest utility of 3D cardiac models in the education of young surgeons who are inexperienced in biventricular repair. The team produced twenty 3D printed heart models with varying congenital heart defects. They concluded that the models provided sufficient detail for the surgeon who had no prior experience in biventricular repair by assisting in the identification of a surgical approach and creating a potential surgical simulation. Patients who underwent surgeries did not experience complications during the surgery, and there was no mortality observed in the one or two years follow-up appointments[21]. Overall, 3D cardiac models is a great educational tool as it lessens the learning curve for surgical trainees, provide a platform for presurgical simulation, and exposure to rare cardiac lesions[18,19].
3D printed cardiac models are not only useful in the planning and surgical repair of cardiac defects. For patients with aortic stenosis, patient-specific 3D printed aortic roots can be used to determine if they are suitable for a transcatheter aortic valve replacement. These aortic root models can undergo physiological testing so that only patients with lower risk of paravalvular leaks, which is associated with a lower late mortality, proceed with the procedure. Cardiac models are also helpful in delineating structures of the left ventricle which is imperative for a patient with hypertrophic cardiomyopathy undergoing septal myomectomy[17]. In patients with heart failure requiring a ventricular assist device (VAD), a cardiac model can be used as a guide to showcase anatomical challenges that can make VAD and cannula placement challenging[17,18].
Three-dimensional cardiac models can be very useful in surgical pre-planning and biventricular repair in complex heterotaxy patients[22,23]. A 3D cardiac model was utilized at our institution for a five-month-old patient with heterotaxy syndrome consisting of atrial situs ambiguous with a common atrium and ipsilateral pulmonary veins, D-looped ventricles, normally related great arteries (ADS), interrupted inferior vena cava with hemi-azygous continuation to the left superior vena cava (SVC) draining into the left and anterior aspect of the common atrium, hepatic veins draining to the right side of the common atrium, a partial AV canal, small anterior muscular VSD[22]. With the aid of a cardiac model [Figures 1 and 2], the cardiothoracic surgeon concluded that intracardiac repair using the Mustard method was not possible due to limited space. The decision was then made to proceed with complex biventricular repair using a modified Warden procedure by creating a baffle starting from the base of the left SVC, incorporating the left and right atrial appendages, and connecting to the right atrium.
Figure 1. Preoperative 3D cardiac model of the five-month-old patient from the apical view demonstrating a common atrium, ipsilateral pulmonary veins and partial AV canal. LPV: Left pulmonary vein; LSVC: left superior vena cava; LV: left ventricle; PA: pulmonary artery; RPV: right pulmonary vein; RV: right ventricle.
Figure 2. Comparison between preoperative 3D cardiac model (A) and postoperative 3D cardiac model (B). Postoperative 3D cardiac model shows a baffle connection between the left superior vena cava and the atrial appendages. LSVC: Left superior vena cava.
Besides benefits in the operating theatre and surgical education, 3D printed heart models can also strengthen relationships as it improves communication among medical providers, and between patient and physician. Cardiologists can utilize 3D printed models to educate patients and their guardians on the progress of their conditions while looking at the pathological region within the heart. Parents of congenital heart disease patients enthusiastically appreciated their encounters with physicians after 3D printed models of the patients’ hearts were used[19,24].
Limitations of 3D printing
Although 3D printed models can accurately depict organ pathology and provide multidirectional views, they still face limitations in areas such as cost, processing time, technicality, etc. The main cost involved is in the initial set-up which can range from USD $5000 to 600,000 for a 3D printer. In addition, a segmentation software can cost up to $15,000 for an annual subscription[17]. However, with the initial set-up complete, the final materials cost is more affordable and can range from less than a hundred to thousands of dollars depending on the complexity of the model, size of the heart, and materials used[25-29]. Despite the high set-up cost of 3D printing, it is still significantly less than the financial and emotional burden on a patient who might require multiple surgeries if the surgery did not go successfully due to the lack of 3D visualization. For simpler pathologies, low-cost printer and material options are available at the expense of processing time and model accuracy[25].
Another limitation of 3D printing is the processing time needed to produce a model, which is dependent on the type of printer used, the complexity of the pathology, and the material used. Powder-based 3D printers can take up to 3 h to finish printing a simple product[17,19,27], but models for patients with complex pathology can take around 7 days to complete[26]. Materials that resemble cardiac myocardium have a higher cost but take less time to produce a cardiac model, whereas less flexible materials have a lower cost but can take up to 10 times longer to print[25]. Hence, despite the benefits of patient-specific anatomy and usefulness in determining surgical plans, long production times can lead to issues like high inpatient hospital bills and psychological anxiety while patients await an appropriate surgical plan, making traditional imaging techniques such as CT or MRI optimal diagnostic methods.
Despite the advancements in imaging technology, flaws can still be present, for example, due to the effect of blood pooling, which results in the inability to fully capture structures like papillary muscles, atrioventricular valves, and semilunar valves. This leads to the production of a 3D model that will be difficult to analyze, diagnose, and plan an appropriate surgery for[19,30]. 3D printed cardiac models are also unable to portray dynamic changes in the heart during cardiac cycles, which is imperative in understanding the pathophysiology of patients with congenital heart diseases[31]. Despite these limitations, some surgeons still prefer 3D cardiac models because the benefits of surgical planning, improved patient communication, and tactile experience of holding the heart can outweigh the costs in most situations.
Ethical issues regarding 3D printing
Despite the undeniable benefits of 3D printing in surgery, there still stand some ethical issues concerning the use of 3D printing to produce patient-specific models. First of all, the use of samples from donors who are not fully competent or who are coerced to give consent is unethical. Therefore, it is best to provide detailed explanations about the risks, benefits, and expected outcomes to the donors and their legal guardians[32]. Currently, materials used for 3D printing can either be obtained synthetically (i.e., polyethylene glycol), from chemically modified natural products (i.e., gelatin methacrylate), or from non-human animal sources. The use of bioink from non-human samples raises another ethical concern because of its potential to cause zoonotic infection in humans. On the contrary, there are institutions and patients that consider the use of human embryonic stem cells unethical. If regulation prevents certain cell lines from being used, it could lead to 3D printing medical tourism in order to get access to these cell lines[32]. In 2017, the Food and Drug Administration (FDA) published a guidance on technical considerations for additive manufacturing, an umbrella term of 3D printing. To protect patient’s privacy, it is advised that device designers maintain proper care of a patient’s personally identifiable information and protected health information. Regarding the strength of the final 3D printed model, the FDA recommends that materials used to produce 3D implants should be noted, and the final product should be tested before surgical use. For example, devices made from metal or ceramic are recommended to undergo mechanical testing if structural inhomogeneity, microstructural voids, or incomplete isolation are detectable[33].
Virtual reality in surgery
VR offers a safe and flexible platform for surgeons to explore a computationally generated patient-specific synthetic world. This includes assessing anatomical regions, generating surgical plans, and familiarizing with procedures to minimize surgical risk[1,34,35]. The possibility to add haptic systems to provide tactile feedback further enhances this experience[1,36]. Furthermore, VR provides physicians with unlimited training opportunities that do not require any patients or cadavers. It can also be used as a form of assessment or a trouble-shooting tool to investigate differing performances between surgeons without putting patients at risk[37].
The creation of virtual reality images also requires medical imaging that captures all aspects of the heart. The imaging data is then segmented, analyzed, and converted from a DICOM file to a file type appropriate for uploading to virtual reality software. Devices such as headsets, controllers, and base stations can be connected to allow the manipulation of virtual images[2,31].
The use of VR in congenital cardiac surgery is mainly for surgical planning and preoperative training. It is especially beneficial in this field given the anatomical variability that can present with each unique patient, thus affecting the approach of the procedure and the extent of repair needed[38,39]. Pushparajah et al.[38] performed a study comparing the understanding of patients with atrioventricular valve lesions and the chosen surgical approach of surgeons that viewed 2D and 3D echocardiograms vs. surgeons that made use of VR images. He concluded that surgeons felt more confident about the pathology and potential surgical approach after viewing images on the VR platform. They also reported that VR was superior to 3D cardiac models since it provided surgeons insight into internal structures like valves. It also allowed visualization of dynamic changes in the heart and depth perception, which can be critical in certain patients[38].
A case about the separation of thoracopagus conjoined twins was described by Juhnke et al.[2], where VR was used to analyze the complex anatomy of the twins and, upon discussion, led to modifications to a more efficient surgical approach. With the aid of VR, the surgeons were able to identify relationships between the two hearts and color code structures that belonged to each twin. This revealed incision depths required to reach the interatrial communication, which was essential to be closed due to its high operative mortality before attempting complete separation of the twins[2].
Ayerbe et al.[40] described a nine-year-old female with DORV and malposition of the great arteries who experienced homograft dysfunction with severe stenosis eight years after undergoing correction by the Rastelli technique. With the use of VR from CT angiography images, they navigated through the complex anatomy, inspected internal structures, and were able to identify the approach for surgical intervention[40].
Ong et al.[41] discussed the use of VR in two patients with congenital heart disease and reported accurate anatomical VR representations compared to intraoperative findings. Knecht et al.[42] reported a case of a 37-year-old male with congenital heart disease and how VR was used to reconstruct the complex heart anatomy from CT scans for pre-procedural planning and guidance of the catheter ablation procedure. VR has also been proven useful in the analysis of patients with sinus venosus defects given its anatomical variants and in investigating the possibility of transcatheter closure with covered stents[39].
Limitations in virtual reality
Like other innovative 3D visualization modalities, the cost of setting up a VR system is up to thousands of dollars and can be a limiting factor. The cost is even higher if haptic feedback devices are factored in. Additionally, there are limitations on both the hardware and software aspects of VR. Some users can find the size, comfort, or battery life of the device unpleasant, and others may get motion-sick from experience. Furthermore, designing surgical simulations to meet the different technical demands of each surgical specialty can be a difficult task[1,5,43,44].
Given that the main usage of VR is in analyzing complex anatomy, presurgical planning, and educational surgical practice, some argue that a formal assessment with defined outcomes needs to be in place to account for the surgeons’ performance before operating on a real patient[5,37]. VR systems should also explore the possibility of assessing the surgeon’s non-technical skills during the procedure, such as communication, situation awareness, and task management skills, which can all affect
Although the option of combining VR with haptic feedback devices is available, some argue that it is still lacking compared to real tactile stimulation like cadavers or 3D printed models. Hence, VR simulation with haptic feedback devices might be more appropriate for the surgical practice of procedures that require limited haptic feedback, such as those involving catheter or wire-based instruments[1].
Lastly, the use of VR is limited to individual use due to the lack of face-to-face communication, but its utility in team-based situations can be further explored[37]. Kim et al.[34] described a study that compared the use of conventional 2D images, non-immersive VR, and fully-immersive VR in the diagnostic accuracy and group discussions of congenital heart diseases among residents and medical students. The study concluded that group discussions using full immersive VR were preferred, and it also led to a higher diagnostic accuracy over the other two imaging modalities[34]. This demonstrates that despite the current limitations of VR, its use in the surgical field can be further explored.
Augmented reality in surgery
AR enhances the real world through interactive elements. Like other 3D imaging modalities, it has become increasingly helpful in assisting surgeons with the identification of anatomical structures to minimize surgical complications and reduce operating hours. Images on augmented reality are created in a similar way to virtual reality, and they mostly differ in the equipment required.
AR has allowed surgeons performing laparoscopic procedures to have a better comprehension of the structures lying beneath the visible organs and the ability to visualize relationships between anatomical structures, overall improving the safety of the procedure and surgical outcomes[11]. In cardiology, AR has been utilized in various minimally invasive applications, and is commonly used in combination with transesophageal echocardiography (TEE). For example, Currie et al.[14] discussed the usage of an AR and TEE guidance system for transcatheter aortic valve implantation deployment in patients with severe aortic stenosis. It is imperative to be accurate in the positioning during the deployment of the stent to prevent complications like embolism or atrioventricular heart block. Furthermore, current procedures usually rely on the use of fluoroscopy with contrast mediums which can increase the risk of acute kidney injury, making the procedure a contraindication in certain patient populations. The integration of AR and TEE as a guidance system addresses these issues by eliminating the use of contrast mediums. It was also reported that procedural errors were lower with the use of the AR guidance system, indicating an overall good valve stent positioning[14].
The improved procedural accuracy from integrating AR and TEE with minimally invasive procedures was also shown in a study involving mitral valve (MV) repair. Ender et al.[45] compared the use of AR and TEE to determine MV annuloplasty size with the current gold standard conventional surgical sizing in 50 patients. Obtaining the right size is essential to avoid complications like mitral regurgitation or mitral stenosis. The team concluded that the AR and TEE system did accurately predict the annuloplasty ring size and anticipated other utility with the system in assisting surgeons with limited experience with MV repair[45]. Also, Chu et al.[15] reported that the addition of AR guidance into MV repair procedures demonstrated a threefold increase in accuracy of the tool navigation and a fivefold decrease in navigation times, leading to overall shorter procedural times with fewer complications. The use of AR in other transcatheter procedures was explored by Liu et al.[16], who further reiterates its positive aspects in assisting with pre-procedural planning, familiarizing with complicated procedures, and as an educational tool for residents and fellows.
Despite data showing the utility of augmented reality in cardiac procedures, its use in congenital heart defects is still limited. Preliminary attempts have been discussed by Opolski et al.[46] in a 30-year-old patient with congenitally corrected transposition of the great arteries and dextrocardia who required transcatheter pacemaker implantation. His congenital heart disease and dextrocardia resulted in altered fluoroscopic orientation, making it technically difficult to identify the optimal location of the pacemaker. However, with the aid of augmented reality, surgeons were able to identify an appropriate location and use it to guide the pacemaker[46].
Limitations of augmented reality
The challenge with implementing AR in surgeries for congenital heart diseases is related to the wide variety of anatomy that patients can present with. Like VR, manipulating simulations to display different intricacies of each patient’s cardiac anatomy can present as a difficult technical task[47]. Additionally, it is also technically difficult to accurately register the heart in congenital heart diseases due to extensive elastic deformation that occurs with respiration and cardiac rhythm. Hence, procedures with few movement and deformation are usually preferred for AR use as it requires less tracking and processing power for the best image resolution[31,48].
As with the other 3D imaging modalities, the use of AR technology is limited due to its high initial set-up cost of up to ten thousand dollars[26]. Furthermore, there is the issue of discomfort due to the size and weight of the head-mounted display after long periods of use. Surgeons should also be aware of a phenomenon called inattentional blindness while using AR, where the ability to identify sudden unexpected objects within the visual field can be affected. They will need to learn how to reduce visual clutter to avoid inattentional blindness and minimize surgical complications[48].
Another issue that resulted in the limited use of AR in surgery is its inability to portray depth perception[31,49]. Several solutions have been devised to address this issue, like light and shade control with rendering techniques, the use of color-coded pictorial depth cues, or the use of stereoscopy. Choi et al.[49] suggested a new system using a combination of AR with VR that will allow manipulation of the images from different angles. This system integrates both imaging modalities in a single window with an aligned view axis to allow seamless switching between imaging modalities. It also allows the input of distance information between the surgical tool and target organ, which further enhances its ability to overcome the depth perception issue during AR use[49].
FUTURE CONSIDERATIONS
The limited use of advanced visualization technologies is likely due to the lack of formal education[47]. Both VR and AR come with a learning curve that can be addressed if there is a proper training program for its use. This program will need to have a standardized evaluation, ways to assess skills retention, and also include fail-safe methods to convert back to the use of traditional imaging modalities if technical issues arise[5,47]. Table 1 compares some of the characteristics to consider for each visualization imaging modality.
Comparison between 3D printing, virtual reality, and augmented reality
3D printing | Virtual reality | Augmented reality | |
Initial set-up costsa | USD $5k to $600k | Up to USD $2k | Up to USD $10k |
Recurring cost | USD $100 to $2kb | Minimal | Minimal |
Processing timec | Hours to days | Minutes to hours | Minutes to hours |
Tactile experience | Present | Absentd | Absent |
Learning curve | Short | Longere | Longere |
Surgical simulation | Single time use | Repeated use | Repeated use |
Environment | Real | Virtual | Real |
Use in real-time surgery | Yes, only as reference | No | Yes |
Team discussions allowed | Yes | Possiblef | Possiblef |
Integration with EMR | No | Possibleg | Possibleg |
Perhaps there is more potential for mixed reality holograms, an evolution of AR, in the field of congenital heart disease. A recent article discussing a 9-year-old boy with DORV, and transposition of the great arteries utilized mixed reality holographic visualization of his cardiac CT angiography images for analysis of his complex anatomy and presurgical planning. Thirty-six congenital heart disease professionals underwent the holographic experience and were asked to identify the anatomical structures, diagnose the disease, come up with a surgical plan, and rate their overall experience. The participants commented that this holographic visualization made comprehending the pathology easier. Furthermore, they were able to come up with a surgical plan that was largely unanimous and rated the overall experience as very positive[50]. Ye et al.[51] agreed with the benefits of mixed reality, and concluded that the modality has higher diagnostic accuracy and shorter surgical planning time compared to a control group using computerized 3D reconstruction of CT angiography images in 34 DORV patients.
The use of mixed reality holograms has also proved its usefulness in telemedicine and long-distance surgical guidance. In an experiment that measured the effectiveness of Microsoft HoloLens in telementoring, both mentor and mentee wore the head-mounted HoloLens and communicated remotely via Skype[52]. Throughout the craniofacial surgical practice, there was a minimal delay in communication between the two participants. The mentor was able to give full instructions while entirely viewing the procedures carried out by the resident through the HoloLens. Most importantly, besides giving voice instruction, both participants could utilize the telestration function to draw lines and arrows on 2D images in the HoloLens[52]. These extended features of AR technology proved it to be a useful tool for telemedicine as it enhances long-distance communication, reduces traveling cost, enriches the knowledge among surgical trainees, and improves patients’ access to experienced surgeons.
CONCLUSION
While some argue that VR and AR are superior to 3D cardiac models because of their magnifying capabilities and reliable resolution that is not limited to the hardware involved, others prefer 3D printed cardiac models because of the tactile stimulation that comes with holding the model in one’s hand. All modalities are also limited by the availability of medical imaging like CT or MRI, reconstruction tools with high initial set-up costs, and logistical difficulties. Despite the cons, all three modalities have proved their usefulness in surgery as they can assist surgeons during pre-procedure planning, minimize postoperative complications, shorten surgical hours, and improve crucial patient-physician relationships. As more preliminary results and emerging evidence demonstrate that the use of these imaging modalities translates to better patient outcomes, surgeons should explore the use of these technologies further and contribute to advancing the surgical field.
DECLARATIONS
Authors’ contributionsContributed to the article and approved the submitted version: Bui I, Bhattacharya A, Wong SH, Agarwal A
Availability of data and materialsNot applicable.
Financial support and sponsorshipNone.
Conflicts of interestAll authors declared that there are no conflicts of interest.
Ethical approval and consent to participateNot applicable.
Consent for publicationNot applicable.
Copyright© The Author(s) 2022.
REFERENCES
1. Bernardo A. Virtual reality and simulation in neurosurgical training. World Neurosurg 2017;106:1015-29.
2. Juhnke B, Mattson AR, Saltzman D, et al. Use of virtual reality for pre-surgical planning in separation of conjoined twins: a case report. Proc Inst Mech Eng H 2019;233:1327-32.
3. de Moraes PH, Olate S, Cantín M, et al. Anatomical reproducibility through 3D printing in cranio-maxillo-facial defects. Int J Morphol 2015;33:826-30.
4. Liu ZJ, Jia J, Zhang YG, Tian W, Jin X, Hu YC. Internal fixation of complicated acetabular fractures directed by preoperative surgery with 3D printing models. Orthop Surg 2017;9:257-60.
5. Cao C, Cerfolio RJ. Virtual or augmented reality to enhance surgical education and surgical planning. Thorac Surg Clin 2019;29:329-37.
6. Low D, Lee CK, Dip LL, Ng WH, Ang BT, Ng I. Augmented reality neurosurgical planning and navigation for surgical excision of parasagittal, falcine and convexity meningiomas. Br J Neurosurg 2010;24:69-74.
8. Zablah JE, Morgan GJ. Innovations in congenital interventional cardiology. Pediatr Clin North Am 2020;67:973-93.
9. Mosso-Vázquez JL, Gao K, Wiederhold BK, Wiederhold MD. Virtual reality for pain management in cardiac surgery. Cyberpsychol Behav Soc Netw 2014;17:371-8.
10. Herron J. Augmented reality in medical education and training. J Electron Resour Med Libr 2016;13:51-5.
11. Kang X, Azizian M, Wilson E, et al. Stereoscopic augmented reality for laparoscopic surgery. Surg Endosc 2014;28:2227-35.
12. Jang J, Tschabrunn CM, Barkagan M, Anter E, Menze B, Nezafat R. Three-dimensional holographic visualization of high-resolution myocardial scar on HoloLens. PLoS One 2018;13:e0205188.
13. Cheng K, Mukherjee P, Curthoys I. Development and use of augmented reality and 3D printing in consulting patient with complex skull base cholesteatoma. Virtual Phys Prototyp 2017;12:241-8.
14. Currie ME, McLeod AJ, Moore JT, et al. Augmented reality system for ultrasound guidance of transcatheter aortic valve implantation. Innovations (Phila) 2016;11:31-9; discussion 39.
15. Chu MW, Moore J, Peters T, et al. Augmented reality image guidance improves navigation for beating heart mitral valve repair. Innovations (Phila) 2012;7:274-81.
16. Liu J, Al'Aref SJ, Singh G, et al. An augmented reality system for image guidance of transcatheter procedures for structural heart disease. PLoS One 2019;14:e0219174.
17. Farooqi KM, Cooper C, Chelliah A, et al. 3D printing and heart failure: the present and the future. JACC Heart Fail 2019;7:132-42.
18. Anwar S, Singh GK, Miller J, et al. 3D printing is a transformative technology in congenital heart disease. JACC Basic Transl Sci 2018;3:294-312.
19. Yoo SJ, Thabit O, Kim EK, et al. 3D printing in medicine of congenital heart diseases. 3D Print Med 2015;2:3.
20. Garekar S, Bharati A, Chokhandre M, et al. Clinical application and multidisciplinary assessment of three dimensional printing in double outlet right ventricle with remote ventricular septal defect. World J Pediatr Congenit Heart Surg 2016;7:344-50.
21. Hoashi T, Ichikawa H, Nakata T, et al. Utility of a super-flexible three-dimensional printed heart model in congenital heart surgery. Interact Cardiovasc Thorac Surg 2018;27:749-55.
22. Betancourt LG, Singh HR, Agarwal A. Partial anomalous left pulmonary artery in heterotaxy syndrome. A case report and review of literature. Prog Pediatr Cardiol 2021;60:101304.
23. Agarwal A. .
24. Milano EG, Capelli C, Wray J, et al. Current and future applications of 3D printing in congenital cardiology and cardiac surgery. Br J Radiol 2019;92:20180389.
25. Lau I, Wong YH, Yeong CH, et al. Quantitative and qualitative comparison of low- and high-cost 3D-printed heart models. Quant Imaging Med Surg 2019;9:107-14.
26. McMenamin PG, Quayle MR, McHenry CR, Adams JW. The production of anatomical teaching resources using three-dimensional (3D) printing technology. Anat Sci Educ 2014;7:479-86.
27. Li KHC, Kui C, Lee EKM, et al. The role of 3D printing in anatomy education and surgical training: a narrative review. MedEdPublish 2016;6:92.
28. Jones DB, Sung R, Weinberg C, Korelitz T, Andrews R. Three-dimensional modeling may improve surgical education and clinical practice. Surg Innov 2016;23:189-95.
29. Valverde I, Gomez G, Gonzalez A, et al. Three-dimensional patient-specific cardiac model for surgical planning in Nikaidoh procedure. Cardiol Young 2015;25:698-704.
30. Ganguli A, Pagan-Diaz GJ, Grant L, et al. 3D printing for preoperative planning and surgical training: a review. Biomed Microdevices 2018;20:65.
31. Goo HW, Park SJ, Yoo SJ. Advanced medical use of three-dimensional imaging in congenital heart disease: augmented reality, mixed reality, virtual reality, and three-dimensional printing. Korean J Radiol 2020;21:133-45.
32. Gilbert F, O'Connell CD, Mladenovska T, Dodds S. Print me an organ? Sci Eng Ethics 2018;24:73-91.
33. Guidance for Industry and Food and Drug Administration Staff. Technical considerations for additive manufactured medical devices. Available from: https://www.fda.gov/media/97633/download [Last accessed on 15 Dec 2021].
34. Kim B, Loke YH, Mass P, et al. A novel virtual reality medical image display system for group discussions of congenital heart disease: development and usability testing. JMIR Cardio 2020;4:e20633.
35. Ayoub A, Pulijala Y. The application of virtual reality and augmented reality in Oral & Maxillofacial Surgery. BMC Oral Health 2019;19:238.
36. Mahmood F, Mahmood E, Dorfman RG, et al. Augmented reality and ultrasound education: initial experience. J Cardiothorac Vasc Anesth 2018;32:1363-7.
37. Pfandler M, Lazarovici M, Stefan P, Wucherer P, Weigl M. Virtual reality-based simulators for spine surgery: a systematic review. Spine J 2017;17:1352-63.
38. Pushparajah K, Chu KYK, Deng S, et al. Virtual reality three-dimensional echocardiographic imaging for planning surgical atrioventricular valve repair. JTCVS Tech 2021;7:269-77.
39. Tandon A, Burkhardt BEU, Batsis M, et al. Sinus venosus defects: anatomic variants and transcatheter closure feasibility using virtual reality planning. JACC Cardiovasc Imaging 2019;12:921-4.
40. Ayerbe VMC, Morales MLV, Rojas CJL, Cortés MLA. Visualization of 3D models through virtual reality in the planning of congenital cardiothoracic anomalies correction: an initial experience. World J Pediatr Congenit Heart Surg 2020;11:627-9.
41. Ong CS, Krishnan A, Huang CY, et al. Role of virtual reality in congenital heart disease. Congenit Heart Dis 2018;13:357-61.
42. Knecht S, Brantner P, Cattin P, Tobler D, Kühne M, Sticherling C. State-of-the-art multimodality approach to assist ablations in complex anatomies-from 3D printing to virtual reality. Pacing Clin Electrophysiol 2019;42:101-3.
43. Chen X, Hu J. A review of haptic simulator for oral and maxillofacial surgery based on virtual reality. Expert Rev Med Devices 2018;15:435-44.
44. Kim Y, Kim H, Kim YO. Virtual reality and augmented reality in plastic surgery: a review. Arch Plast Surg 2017;44:179-87.
45. Ender J, Koncar-Zeh J, Mukherjee C, et al. Value of augmented reality-enhanced transesophageal echocardiography (TEE) for determining optimal annuloplasty ring size during mitral valve repair. Ann Thorac Surg 2008;86:1473-8.
46. Opolski MP, Michałowska IM, Borucki BA, Nicińska B, Szumowski Ł, Sterliński M. Augmented-reality computed tomography-guided transcatheter pacemaker implantation in dextrocardia and congenitally corrected transposition of great arteries. Cardiol J 2018;25:412-3.
47. Salavitabar A, Figueroa CA, Lu JC, Owens ST, Axelrod DM, Zampi JD. Emerging 3D technologies and applications within congenital heart disease: teach, predict, plan and guide. Future Cardiol 2020;16:695-709.
48. Vávra P, Roman J, Zonča P, et al. Recent development of augmented reality in surgery: a review. J Healthc Eng 2017;2017:4574172.
49. Choi H, Cho B, Masamune K, Hashizume M, Hong J. An effective visualization technique for depth perception in augmented reality-based surgical navigation. Int J Med Robot 2016;12:62-72.
50. Brun H, Bugge RAB, Suther LKR, et al. Mixed reality holograms for heart surgery planning: first user experience in congenital heart disease. Eur Heart J Cardiovasc Imaging 2019;20:883-8.
51. Ye W, Zhang X, Li T, Luo C, Yang L. Mixed-reality hologram for diagnosis and surgical planning of double outlet of the right ventricle: a pilot study. Clin Radiol 2021;76:237.e1-7.
Cite This Article
Export citation file: BibTeX | RIS
OAE Style
Bui I, Bhattacharya A, Wong SH, Agarwal A. Role of advanced three-dimensional visualization modalities in congenital heart surgery. Vessel Plus 2022;6:31. http://dx.doi.org/10.20517/2574-1209.2021.97
AMA Style
Bui I, Bhattacharya A, Wong SH, Agarwal A. Role of advanced three-dimensional visualization modalities in congenital heart surgery. Vessel Plus. 2022; 6: 31. http://dx.doi.org/10.20517/2574-1209.2021.97
Chicago/Turabian Style
Bui, Ivy, Arunabh Bhattacharya, Si-Hui Wong, Arpit Agarwal. 2022. "Role of advanced three-dimensional visualization modalities in congenital heart surgery" Vessel Plus. 6: 31. http://dx.doi.org/10.20517/2574-1209.2021.97
ACS Style
Bui, I.; Bhattacharya A.; Wong S.H.; Agarwal A. Role of advanced three-dimensional visualization modalities in congenital heart surgery. Vessel Plus. 2022, 6, 31. http://dx.doi.org/10.20517/2574-1209.2021.97
About This Article
Special Issue
Copyright
Data & Comments
Data
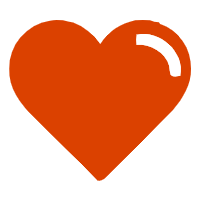

Comments
Comments must be written in English. Spam, offensive content, impersonation, and private information will not be permitted. If any comment is reported and identified as inappropriate content by OAE staff, the comment will be removed without notice. If you have any queries or need any help, please contact us at support@oaepublish.com.