Is insulin pro-atherogenic at the cellular level?
Abstract
Aim: This study was undertaken to test the hypothesis that insulin treatment has unexpected pro-atherogenic effects at the cellular level, namely, proliferative activity and intracellular cholesterol content.
Methods: Primary cultures of subendothelial cells derived from non-atherosclerotic human aorta and mouse peritoneal macrophages were used to investigate the in vitro effect of insulin on atherosclerosis-related parameters, such as cellular cholesterol content and proliferation rate. Additionally, the effect of insulin treatment in 33 type 1 diabetic patients on serum atherogenicity (i.e. its ability to induce cholesterol accumulation in cultured cells) was investigated.
Results: Insulin (1-1,000 µU/mL) did not affect [3H]-thymidine incorporation or cholesterol content in either type of cultured cell. Most blood sera obtained from type 1 diabetic patients induced a 1.5- to 1.7-fold increase in cholesterol content of cultured cells, but this effect did not correlate with serum insulin levels. Exogenous insulin added to cultured cells did not modify the effect of patient's sera on cholesterol level and proliferation of cultured cells.
Conclusion: The results suggest that insulin does not exert direct atherogenic actions at the level of arterial cells, with the respect to proliferative activity and cholesterol content.
Keywords
Introduction
Insulin-dependent (type 1) diabetes mellitus is known to favor an accelerated development of atherosclerosis, although the reasons for premature atherogenesis in diabetic patients remain obscure. Several hypotheses exist, which try to describe the molecular, cellular and biochemical mechanisms of premature atherogenesis in diabetic patients, but all have failed to sufficiently explain this phenomenon. Proposed mechanisms include the harmful effects of advanced glycosylation end products, modification of low density lipoproteins by non-enzymatic glycosylation, lipoprotein retention in the arterial wall, enhanced oxidative stress, inflammation, endothelial dysfunction, and alterations in metabolism of vitamins and minerals[1-5]. In addition to these potential factors, it is necessary to consider the possible role of insulin in the process of atherosclerosis development. Since hyperinsulinemia is associated with increased cardiovascular risk, hyperinsulinemia and hepatic portal hypoinsulinemia due to injection of insulin preparations widely used in the treatment of type 1 diabetic patients could be responsible, at least in part, for cardiovascular consequences of the disease. The results of epidemiological studies and experimental studies in animal models supported systemic hyperinsulinemia as a major plausible factor in the development of atherosclerosis in diabetic patients[6-10]. Insulin resistance is strongly associated with hyperinsulinemia, and is considered as the major pathologic mechanism for susceptibility to premature atherosclerosis and cardiovascular disease[11,12]. However, the effects of exogenous insulin on atherosclerosis progression have not been sufficiently studied. Experimental studies demonstrate direct in vitro effects of insulin on angiogenesis, as well as on endothelial and arterial smooth muscle cell proliferation[13,14]. Nevertheless, this contention remains controversial, and there is no definitive settlement on the role of insulin in atherogenesis[15-17]. To elucidate this matter, the present study tested the influence of insulin on cellular proliferation and total cholesterol content in cultured subendothelial cells derived from human aorta and mouse peritoneal macrophages. Additionally, the effect of exogenous insulin on atherogenicity of serum from diabetic patients was investigated.
Methods
Patients
This study was conducted in accordance with the Helsinki Declaration of 1975 as revised in 1983. It was approved by the local ethics committee of the Institute for Atherosclerosis Research, Skolkovo Innovation Center, Moscow, Russia. All participants gave their written informed consent prior to their inclusion in the study.
The group of study participants consisted of 33 type 1 diabetic patients aged 20 to 44 (17 men, 16 women) with diabetes duration ranging from 0 to 16 years. They were characterized by rather low level of antibodies to insulin (less than 50 nU/mL), therefore, the possibility of insulin resistance attributable to antibodies was excluded[18]. The type of diabetes was verified according to recommendations from the American Diabetes Association[19]. None of the patients had clinical manifestations of coronary heart disease. All patients received intensive insulin therapy with glargine ("Lantus", Sanofi-Aventis Deutschland GmbH, Germany) as basal insulin, and glulisine ("Apidra", Sanofi-Aventis Deutschland GmbH, Germany) as prandial insulin. None of the patients had coronary heart disease or other clinical manifestations of atherosclerosis, arterial hypertension or kidney disease. The selection and recruitment of study participants was designed to avoid any significant comorbidity or drug administration, which could potentially affect the results of experiments. Venous blood (5 mL) was drawn once in the morning before meals at the first day at the hospital. Fasting blood glucose was 8.2 ± 0.4 mmol/L, HbA1c was 9.2 ± 0.3%, serum cholesterol level was 5.2 ± 0.2 mmol/L, and triglyceride level was 1.4 ± 0.2 mmol/L.
Additionally, the second group of study participants consisted of 3 patients with newly diagnosed type 1 diabetes mellitus (1 man and 2 women aged 18, 19 and 23, respectively), who were characterized by low levels of immunoreactive insulin (IRI) and residual C-peptide secretion (1.6 ± 0.5 mU/L and 0.8 ± 0.2 ng/mL, respectively), and significantly elevated blood glucose levels (20.0 ± 0.2 mmol/L) without severe ketoacidosis on admission to hospital. Intensive therapy with insulin glulisine and insulin glargine was started immediately. For monitoring of glucose level, IRI level and blood serum atherogenicity, venous blood was taken several times throughout the duration of hospitalization immediately before the start of intensive insulin therapy, then at 30 min, 2 h, 6 h, 12 h, 24 h and 7 days after the first insulin injection. On the 10th day, the patients were discharged from the hospital and were examined at outpatient clinics 21 days after the start of intensive insulin therapy.
Blood collected from patients was incubated for 1 h at 37 ºC and centrifuged for 30 min at 1,000 g. The resultant serum samples were stored frozen (-20 ºC) for 4-7 days before examination.
Reagents
Medium 199, fetal calf serum (FCS), penicillin, streptomycin, Fungizone and L-glutamine were purchased from GIBCO Europe (Paisley, UK). Collagenase type II was obtained from Worthington Diagnostic System (Freehold, UK). [6-3H]-thymidine (21 Ci/mmol) was purchased from Amersham International (Amersham, UK). Assay kits for total cholesterol determination were from Cell Biolabs, Inc. (San Diego, CA, USA). Other reagents were purchased from Sigma-Aldrich Co., LLC (St. Louis, MO, USA).
Cell culture
To study the direct effects of insulin on intracellular cholesterol accumulation and cellular proliferation, cells were isolated from the non-atherosclerotic sub-endothelial (elastico-hyperplastic) sublayer of the intima by digestion of human aortic tissue with 0.15% collagenase under sterile conditions, as described elsewhere[20]. The autopsy material was taken from subjects aged 50 to 58 who had died suddenly by accident. After digestion, enzyme-isolated cells were separated from the remaining tissue by filtering through nylon mesh, and subsequently resuspended in Medium 199 containing standard additives: 2 mmol/L L-glutamine, 100 U/mL penicillin, 100 µg/mL streptomycin, 2.5 µg/mL Fungizone and 10% of FCS. Cells were seeded into 48-well or 24-well tissue culture plates at a density of (3-4) × 104 cells/cm2 of growth area. The cells were cultured at 37 ºC in a humidified CO2-incubator (95% air and 5% CO2). The primary cultures contained a mixed cell population made up primarily (95%) of typical and modified smooth muscle cells as it was earlier defined by the ultrastructural features and immunofluorescent markers. The medium was changed each day. On the 7th day in culture, Medium 199 containing 40% blood serum from type 1 diabetic patients, 1 µCi/mL [3H]-thymidine and the indicated concentrations of insulin (0.001-1,000 mU/mL), or Medium 199 containing 10% of FCS, 1 µCi/mL [3H]-thymidine and insulin (0.001-1,000 mU/mL) were applied to cell cultures. After 24 h incubation, cells were rinsed 3 times with phosphate buffered saline (PBS), 3 times with PBS containing 0.2% bovine serum albumin, and again 3 times with PBS. Cellular lipids were extracted with the mixture of n-hexane and isopropanol (3:2, vol:vol) as described elsewhere[20]. Total cholesterol content was determined enzymatically using commercial assay kits. After delipidation, the cells were rinsed 3 times with 5% trichloroacetic acid and then were dissolved in 0.1 mol/L NaOH. Aliquots were used for cellular protein determination and [3H]-thymidine incorporation as described by Tertov et al.[21].
To measure serum atherogenicity (i.e. its ability to induce cholesterol accumulation in cultured cells), BALB/c mouse resident peritoneal macrophages were taken from non-stimulated animals as described by Adams[22]. Afterward, 2 × 105 cells were seeded into each well of 24-well plates in Medium 199 containing antibiotics and 10% of FCS. After a 4-h incubation, the cultures were rinsed with Medium 199 to remove unattached cells. Then cells were incubated for additional 4 h in Medium 199 containing 10% of examined patient’s serum. Lipid extraction, cellular protein and cholesterol measurements were performed as described above.
Statistical analysis
Results are reported as mean ± SEM, based on triplicate measurements in each of 4 independent experiments. Significance of differences was evaluated using the IBM SPSS 20.0 statistical program package and was assumed for P values < 0.05.
Results
Twenty-five, or 70% of 33 type 1 diabetic patient’s sera exhibited an atherogenic potential, i.e. increased total cholesterol content of cultured mouse peritoneal macrophages by 50-70% as compared to control. This effect did not depend on patient’s age, gender, plasma lipid levels, or diabetes duration. Moreover, serum-induced cholesterol accumulation did not correlate with IRI level in tested serum samples (r = -0.09, P > 0.6).
In those 3 patients with newly diagnosed type 1 diabetes mellitus, blood serum obtained immediately before the beginning of intensive insulin therapy induced significant cholesterol accumulation in cultured mouse peritoneal macrophages [Table 1]. As it was expected, after the start of intensive insulin therapy, IRI level increased rapidly, whereas C-peptide level remained low (the data not shown). By the end of the 1st day of intensive insulin therapy, blood glucose decreased to 10.5 ± 0.8 mmol/L, and IRI level accounted for 28 ± 3 mU/L. Further treatment resulted in the achievement of satisfactory glycemic control (data not shown). Along with these changes, the atherogenic effect of serum stayed practically invariable up to the 21st day of intensive insulin therapy, despite the steady decrease in blood glucose and elevation in IRI level [Table 1]. Therefore, the ability of diabetic blood serum to induce intracellular cholesterol accumulation was not directly associated with either plasma insulin concentration or the state of glycemic control.
The effect of insulin therapy in newly diagnosed type 1 diabetic patients on plasma IRI level and blood serum atherogenicity
Time | IRI level (mU/L) | Intracellular cholesterol accumulation, % of control (*) |
---|---|---|
Patient 1 | ||
Before insulin therapy | 1.7 | 200 ± 8 |
30 min | 8 | 205 ± 6 |
2 h | 10 | 195 ± 7 |
6 h | 13 | 202 ± 5 |
12 h | 27 | 186 ± 6 |
24 h | 23 | 180 ± 9 |
7 days | 29 | 195 ± 8 |
21 days | 35 | 180 ± 9 |
Patient 2 | ||
Before insulin therapy | 0.8 | 160 ± 7 |
30 min | 6 | 162 ± 8 |
2 h | 11 | 168 ± 3 |
6 h | 15 | 160 ± 7 |
12 h | 30 | 164 ± 5 |
24 h | 34 | 170 ± 6 |
7 days | 20 | 164 ± 7 |
21 days | 27 | 169 ± 6 |
Patient 3 | ||
Before insulin therapy | 2.4 | 220 ± 9 |
30 min | 7.8 | 219 ± 6 |
2 h | 10 | 211 ± 4 |
6 h | 15 | 208 ± 6 |
12 h | 25 | 219 ± 4 |
24 h | 28 | 209 ± 5 |
7 days | 50 | 199 ± 5 |
21 days | 52 | 214 ± 5 |
We have also studied the direct effect of insulin on cholesterol content and proliferative activity in cultured human aortic intima cells [Table 2] and mouse peritoneal macrophages. At various concentrations (1-1,000 mU/mL), insulin had no effect on intracellular cholesterol content. Moreover, insulin did not stimulate proliferative activity of cultured intimal cells; slight deviations seemed to be random and non-systematic [Table 2]. Additionally, the lack of insulin effect on intracellular cholesterol content and [3H]-thymidine incorporation was registered when extremely low (0.001, 0.01 and 0.1 mU/mL) insulin concentrations were used (data not shown). Similar results were obtained in cultured mouse peritoneal macrophages (data not shown).
The effect of insulin on [3H]-thymidine incorporation and total cholesterol content of cultured intimal human cells
Insulin concentration(mU/mL) | Intracellular total cholesterol content (μg/mg cell protein) | [3H]-thymidine incorporation (dpm/μg cell protein) |
---|---|---|
Control | 39 ± 2 | 36 ± 2 |
1 | 40 ± 1 | 40 ± 2 |
10 | 40 ± 2 | 38 ± 1 |
100 | 40 ± 1 | 34 ± 1 |
1,000 | 38 ± 2 | 39 ± 3 |
The atherogenic effects of sera randomly taken from four type 1 diabetic patients were further studied using the primary culture of human intimal aortic cells. These studies revealed that the sera taken from 2 patients induced a significant increase in total cholesterol content of cultured human intimal cells, while 2 other serum samples proved to be non-atherogenic, i.e. did not produce the changes in intracellular cholesterol as compared to control cells incubated in the absence of human serum [Table 3]. Insulin addition at concentrations of 1-1,000 mU/mL to the cultured cells did not affect serum atherogenicity [Table 3]. One of the sera induced enhanced [3H]-thymidine incorporation by cultured cells along with pronounced cholesterol accumulation. Insulin addition did not modify this effect. Similarly, no significant changes in [3H]-thymidine incorporation were observed in cells incubated with 3 other serum samples, even when insulin was used in high concentrations [Table 3]. When these serum samples were tested on cultured mouse peritoneal macrophages, similar results were obtained with respect to either cellular cholesterol content or [3H]-thymidine incorporation (data not shown).
The effect of exogenous insulin addition on atherogenic properties of diabetic patients' serum
Intracellular cholesterol content, μg/mg cell protein at insulinconcentration (mU/mL) | [3H]-thymidine incorporation, dpm/mg cell protein at insulinconcentration (mU/mL) | |||||||||
---|---|---|---|---|---|---|---|---|---|---|
0 | 1 | 10 | 100 | 1,000 | 0 | 1 | 10 | 100 | 1,000 | |
Control | 43 ± 1 | 45 ± 1 | 42 ± 2 | 45 ± 1 | 44 ± 2 | 16 ± 3 | 19 ± 2 | 17 ± 1 | 19 ± 2 | 20 ± 2 |
Patient 4 | 43 ± 3 | 45 ± 2 | 46 ± 2 | 44 ± 2 | 47 ± 3 | 16 ± 3 | 12 ± 4 | 16 ± 5 | 19 ± 6 | 20 ± 2 |
Patient 5 | 44 ± 3 | 45 ± 2 | 47 ± 2 | 47 ± 2 | 46 ± 3 | 18 ± 3 | 15 ± 2 | 16 ± 1 | 13 ± 3 | 14 ± 3 |
Patient 6 | 69 ± 3* | 69 ± 5* | 71 ± 4* | 74 ± 3* | 74 ± 4* | 12 ± 2 | 15 ± 2 | 15 ± 4 | 17 ± 2 | 18 ± 5 |
Patient 7 | 82 ± 4* | 81 ± 2* | 86 ± 3* | 86 ± 3* | 81 ± 5* | 27 ± 3# | 28 ± 3# | 35 ± 4# | 34 ± 4# | 30 ± 3# |
Discussion
Enhanced cellular proliferative activity and deposition of intracellular lipids in the vessel wall, mainly free and esterified cholesterol are typical features of early atherosclerotic lesions. Previous studies demonstrated that blood sera or low-density lipoprotein from most of type 1 diabetic patients, unlike healthy subjects’ sera, are able to induce cholesterol deposition in cultured macrophages and human intimal cells[20,23-27]. However, one cannot rule out the possibility that some other factors may play a role. Hyperinsulinemia is thought to be an independent risk factor for atherosclerosis, as was demonstrated earlier in numerous epidemiologic studies[10,28-31]. On the other hand, most of the data were obtained on groups of non-diabetic individuals or type 2 diabetic patients. At the same time, type 1 diabetes is characterized by elevated insulin levels due to exogenous insulin administration, but the role of insulin in atherogenesis in this category of patients remains unknown.
Theoretically, there are many reasons why insulin might affect the vessel wall in diabetic patients. Insulin is a widely recognized and important growth factor. Initially, the hypothesis on insulin atherogenicity derived from old studies in non-diabetic animals given large doses of insulin, which showed enhanced cholesterol synthesis in the aorta[32-34]. Later studies showed that atherosclerosis-like biochemical and histological changes in aortas of Wistar rats could be induced by long-term insulin treatment and hyperinsulinemia[35,36]. However, these findings were not supported in other studies in hyperinsulinemic or insulin-treated animals[37,38]. Insulin did not produce any effect on the transfer of cholesterol from circulation into arterial wall[39]. It was also demonstrated that insulin did not modify lipogenesis by the rat intima-media even at very high concentrations[40].
It is suggested that insulin may cause an increased cellular proliferation in tissue culture[13,41]. However, to demonstrate such an effect of insulin, the cells needed to be starved in serum-deficient medium for several days, the conditions being far from physiological, and then only at high insulin concentrations[42]. However, Ledet had not been able to demonstrate the growth-stimulating effect of insulin; moreover, there was a growth-promoting effect of serum from recent-onset type 1 diabetic patients, although serum insulin levels were extremely low[43]. Also, against the "insulin hypothesis" stand the observations of beneficial effects of insulin treatment on the development of atherosclerosis-like lesions in streptozotocin-diabetic rats. In well-controlled rats, tunica media of coronary arteries contained the normal relative amount of connective tissue and number of cells, as compared to the poorly controlled diabetic animals[44]. Additionally, hyperinsulinemia did not stimulate the early stages of arterial smooth muscle cells proliferation in the rat after aortic injury[45]. So, the speculated pro-atherogenic effects of insulin were intensively discussed for a long time, but then left aside for decades, without reaching consensus.
All further studies focused on clinical effectiveness of insulin therapy, with a partial respect to cardiovascular outcomes, which could serve as the indirect markers of proatherogenic or, vice versa, beneficial effects of insulin on atherosclerosis progression. Large observational studies performed in diabetic patients did not solve the controversy on the effects of insulin treatment on atherosclerosis progression and cardiovascular risk, although they have demonstrated some reduction in cardiovascular events under improved glycemic control during the long-term follow-up[46]. The Epidemiology of Diabetes Interventions and Complications (EDIC) Study, which was designed as the follow-up long-term study of the Diabetes Control and Complications Trial (DCCT), was aimed to estimate the effects of glycemic control on major cardiovascular endpoints (all fatal and nonfatal cardiovascular events, angina, revascularization, and also fatal and nonfatal myocardial infarction, and stroke as secondary endpoints) in type 1 diabetes mellitus patients[47,48]. The EDIC study participants were followed annually, and after a mean of 18 years from the start of the DCCT, a 42% reduction in cardiovascular disease outcomes was observed along with a 57% reduction in fatal and nonfatal myocardial infarction, and stroke[48]. Thus, prospective studies have provided indirect evidence supporting the absence of harmful effects of intensive insulin therapy on atherosclerosis progression in type 1 diabetic patients. Moreover, intensive insulin therapy was shown to be beneficial with respect to instrumental measures of atherosclerosis, namely, coronary artery calcification and carotid intima media thickness[49,50].
In contrast, studies in type 2 diabetic patients who were appointed to intensive insulin therapy provided rather controversial results. The United Kingdom Prospective Diabetes Study (UKPDS) demonstrated a non-significant 16% decrease in fatal and nonfatal myocardial infarction along with a non-significant 6% decrease in all-cause mortality in newly diagnosed type 2 diabetic patients followed-up for 10 years[51]. Post-trial follow-up for another 10 years demonstrated 15% reduction in myocardial infarction, and 13% reduction in all-cause mortality[52]. However, it is difficult to interpret the UKPDS results, since intensive glycemic control was achieved by sulfonylurea derivatives and/or insulin. Later studies mainly aimed to assess cardiovascular disease risk in intensively controlled type 2 diabetic patients [Action to Control Cardiovascular Risk in Diabetes (ACCORD); Action in Diabetes and Vascular Disease: Preterax and Diamicron Modified-Release Controlled Evaluation (ADVANCE); Veterans Affairs Diabetes Trial (VADT)] failed to reproduce the beneficial effects observed in UKPDS[53-55]. It should be noted that insulin use ranged from 41-90% among intensively controlled subjects in these trials. Within 3.5-5.6 years of follow-up, no significant reductions in cardiovascular disease were demonstrated. Moreover, the ACCORD trial was prematurely stopped after a median follow-up of 3.4 years due to a statistically significant 22% higher all-cause mortality just in a subgroup with intensive glucose control[56]. Thus, it could be proposed that intensive glucose lowering in treatment of type 2 diabetic patients is associated with a higher incidence of mortality, although the role of insulin treatment was never elucidated. However, the most recent meta-analysis of the benefits and harms of intensive glucose lowering therapy performed on the data from 58,160 type 2 diabetic patients in 13 randomized controlled trials has provided the evidence that intensive glucose lowering therapy compared to conventional glucose control therapy is associated with a reduced risk of major cardiovascular events and myocardial infarction. At the same time, intensive glucose lowering therapy does not affect significantly the risks of cardiac death, stroke, congestive heart failure, and total mortality[57].
The results of our study show that insulin does not exert direct atherogenic actions, at least on such phenotypic characteristics as intracellular cholesterol accumulation and cell proliferation. An effect on cholesterol content or proliferative activity was not observed in cultured cells, a result that could possibly be attributed to the high concentration of serum used. Certainly, these results do not indicate that insulin has no proatherogenic effect at all, and numerous possibilities of atherogenic action remain, which could be detected by other parameters. On the other hand, blood serum atherogenicity in type 1 diabetic patients did not correlate with insulin level in serum samples. The addition of exogenous insulin to the cultural medium did not modify atherogenic effects of diabetic patients’ sera. The obtained results are in good agreement with the data on the absence of atherogenicity of insulin from studies performed on experimental models different from ours[37-40,44,45].
It must be noted that this study has certain limitations. First, the power of the study may be limited because of the small sample size. Second, the second group of patients with newly diagnosed type 1 diabetes mellitus consisted only of 3 patients, that is too low for complex disease; therefore, the obtained results may be discussed only as a case report. Third, only 2 major traits of atherosclerotic cellular phenotype (namely, cholesterol accumulation and proliferative activity) were examined, and others were not studied, like enhanced synthesis of protein and matrix components, and proinflammatory response. Finally, insulin action in cell culture studies was not controlled in experiments using insulin receptor knockout/knockdown cells.
Biologically, from a functional point of view, insulin overlaps with various much more potent growth factors, e.g. somatomedins, platelet-derived growth factor and epidermal growth factor, which may disguise the action of insulin, especially in epidemiological surveys. So, one may speculate that hyperinsulinemia in diabetic patients might play an atherogenic role but in indirect manner or be tightly accompanied by other atherogenic factors, which are responsible for the initiation of atherogenesis and further development of atherosclerotic lesions.
Declarations
Authors’ contributionsConcept and experimental studies, data analysis and statistical analysis, and manuscript editing: I.A. Sobenin
Literature search and patients’ recruitment: V.A. Orekhova
Clinical examination and clinical data acquisition: A.V. Grechko
General coordination and supervision of the research project, and manuscript editing: A.N. Orekhov
Financial support and sponsorshipThe study was supported by the Ministry of Education and Sciences of the Russian Federation (Grant # 14.W02.16.6995-Scientific_School).
Conflict of interestsThere are no conflicts of interests.
Patient consentAll participants gave their written informed consent prior to their inclusion in the study.
Ethics approvalThis study was kept in accordance with the Helsinki Declaration of 1975 as revised in 1983. It was approved by the local ethics committee of the Institute for Atherosclerosis Research, Skolkovo Innovation Center, Moscow, Russia.
Copyright© The Author(s) 2017.
REFERENCES
1. Yahagi K, Kolodgie FD, Lutter C, Mori H, Romero ME, Finn AV, Virmani R. Pathology of human coronary and carotid artery atherosclerosis and vascular calcification in diabetes mellitus. Arterioscler Thromb Vasc Biol 2017;37:191-204.
2. Heier M, Margeirsdottir HD, Gaarder M, Stensæth KH, Brunborg C, Torjesen PA, Seljeflot I, Hanssen KF, Dahl-Jørgensen K. Soluble RAGE and atherosclerosis in youth with type 1 diabetes: a 5-year follow-up study. Cardiovasc Diabetol 2015;14:126.
3. Hunt KJ, Baker NL, Cleary PA, Klein R, Virella G, Lopes-Virella MF; DCCT/EDIC Group of investigators. Longitudinal association between endothelial dysfunction, inflammation, and clotting biomarkers with subclinical atherosclerosis in type 1 diabetes: an evaluation of the DCCT/EDIC cohort. Diabetes Care 2015;38:1281-9.
4. Yoon HJ, Lee YH, Kim SR, Rim TH, Lee EY, Kang ES, Cha BS, Lee HC, Lee BW. Glycated albumin and the risk of micro- and macrovascular complications in subjects with type 1 diabetes. Cardiovasc Diabetol 2015;14:53.
5. Heier M, Margeirsdottir HD, Brunborg C, Hanssen KF, Dahl-Jørgensen K, Seljeflot I. Inflammation in childhood type 1 diabetes; influence of glycemic control. Atherosclerosis 2015;238:33-7.
6. Zeadin MG, Petlura CI, Werstuck GH. Molecular mechanisms linking diabetes to the accelerated development of atherosclerosis. Can J Diabetes 2013;37:345-50.
7. Shah MS, Brownlee M. Molecular and cellular mechanisms of cardiovascular disorders in diabetes. Circ Res 2016;118:1808-29.
9. Tune JD, Goodwill AG, Sassoon DJ, Mather KJ. Cardiovascular consequences of metabolic syndrome. Transl Res 2017;183:57-70.
11. Gwinup G, Elias AN. Hypothesis: insulin is responsible for the vascular complications of diabetes. Med Hypotheses 1991;34:1-6.
12. Bansilal S, Farkouh ME, Fuster V. Role of insulin resistance and hyperglycemia in the development of atherosclerosis. Am J Cardiol 2007;99:B6-14.
13. Stout RW. Insulin as a mitogenic factor: role in the pathogenesis of cardiovascular disease. Am J Med 1991;90:S62-5.
14. Escudero CA, Herlitz K, Troncoso F, Guevara K, Acurio J, Aguayo C, Godoy AS, González M. Pro-angiogenic role of insulin: from physiology to pathology. Front Physiol 2017;8:204.
15. Rask-Madsen C, Buonomo E, Li Q, Park K, Clermont AC, Yerokun O, Rekhter M, King GL. Hyperinsulinemia does not change atherosclerosis development in apolipoprotein E null mice. Arterioscler Thromb Vasc Biol 2012;32:1124-31.
18. Radermecker RP, Renard E, Scheen AJ. Circulating insulin antibodies: influence of continuous subcutaneous or intraperitoneal insulin infusion, and impact on glucose control. Diabetes Metab Res Rev 2009;25:491-501.
19. American Diabetes Association. (2) Classification and diagnosis of diabetes. Diabetes Care 2015;38 Suppl:S8-16.
20. Sobenin IA, Galitsyna EV, Grechko AV, Orekhov AN. Small dense and desialylated low density lipoprotein in diabetic patients. Vessel Plus 2017;1:29-37.
21. Tertov VV, Orekhov AN, Ryong LH, Smirnov VN. Intracellular cholesterol accumulation is accompanied by enhanced proliferative activity of human aortic intimal cells. Tissue Cell 1988;20:849-54.
22. Adams DO. Macrophages. In: Jakoby WB, Pastan JA, editors. Methods in Enzymology. New York: Academic Press; 1979. pp. 494-506.
23. Slavina ES, Madanat AYa, Pankov YuA, Syrkin AL, Tertov VV, Orekhov AN. Diabetes mellitus and atherosclerosis. N Engl J Med 1987;317:836.
24. Sobenin IA, Tertov VV, Koschinsky T, Bünting CE, Slavina ES, Dedov II, Orekhov AN. Modified low density lipoprotein from diabetic patients causes cholesterol accumulation in human intimal aortic cells. Atherosclerosis 1993;100:41-54.
25. Sobenin IA, Tertov VV, Orekhov AN. Atherogenic modified LDL in diabetes. Diabetes 1996;45:S35-9.
26. Lyons TJ, Klein RL, Baynes JW, Stevenson HC, Lopes-Virella MF. Stimulation of cholesteryl ester synthesis in human monocyte-derived macrophages by low-density lipoproteins from type 1 (insulin-dependent) diabetic patients: the influence of non-enzymatic glycosylation of low-density lipoproteins. Diabetologia 1987;30:916-23.
27. Lopes-Virella MF, Virella G. Pathogenic role of modified LDL antibodies and immune complexes in atherosclerosis. J Atheroscler Thromb 2013;20:743-54.
29. Savage PJ, Saad MF. Insulin and atherosclerosis: villain, accomplice, or innocent bystander? Br Heart J 1993;69:473-5.
30. Fontbonne AM, Eschwège EM. Insulin and cardiovascular disease. Paris prospective study. Diabetes Care 1991;14:461-9.
31. Yosefy C. Hyperglycaemia and its relation to cardiovascular morbidity and mortality: has it been resolved? Acta Diabetol 2003;40:S380-8.
33. Wahlqvist ML, Stout RW. The effect of insulin on the incorporation of sodium (1-14C)-acetate into the lipids of the rat aorta. Diabetologia 1972;8:305-6.
35. Sato Y, Shiraishi S, Oshida Y, Ishiguro T, Sakamoto N. Experimental atherosclerosis-like lesions induced by hyperinsulinism in Wistar rats. Diabetes 1989;38:91-6.
36. Abe H, Bandai A, Makuuchi M, Idezuki Y, Nozawa M, Oka T, Osuga J, Watanabe Y, Inaba T, Yamada N. Hyperinsulinaemia accelerates accumulation of cholesterol ester in aorta of rats with transplanted pancreas. Diabetologia 1996;39:1276-83.
37. Chattopadhyay DP, Martin JM. Effect of insulin on the in vitro synthesis of sterol and fatty acid by aorta and liver from diabetic rats. J Atheroscler Res 1969;10:131-4.
38. Chobanian AV, Gerritsen GC, Brecher PI, McCombs L. Aortic glucose metabolism in the diabetic Chinese hamster. Diabetologia 1974;10 Suppl:589-93.
39. Christensen S, Jensen J. Uptake of labelled cholesterol from plasma by aortic intima-media in control and insulin-injected rabbits. J Atheroscler Res 1965;5:258-9.
40. Capron L, Jarnet J. Effects of injury and insulin on lipid synthesis from glucose by the rat thoracic aorta. Arterioscler Thromb 1991;11:91-6.
41. Pfeifle B, Ditschuneit HH, Ditschuneit H. Insulin as a cellular growth regulator of rat arterial smooth muscle cells in vitro. Horm Metab Res 1980;12:381-5.
42. King GL, Buzney SM, Kahn CR, Hetu N, Buchwald S, Macdonald SG, Rand LI. Differential responsiveness to insulin of endothelial and support cells from micro- and macrovessels. J Clin Invest 1983;71:974-9.
43. Ledet T. Growth hormone stimulating the growth of arterial medial cells in vitro. Absence of effect of insulin. Diabetes 1976;25:1011-7.
44. Baandrup U, Ledet T, Rasch R. Experimental diabetic cardiopathy preventable by insulin treatment. Lab Invest 1981;45:169-73.
45. Ridray S, Ktorza A, Picon L, Capron L. In vivo effect of insulin on the acute proliferative response of the rat aorta to injury. Arterioscler Thromb 1992;12:633-8.
46. Joseph JJ, Donner TW. Long-term insulin glargine therapy in type 2 diabetes mellitus: a focus on cardiovascular outcomes. Vasc Health Risk Manag 2015;11:107-16.
47. Nathan DM, Genuth S, Lachin J, Cleary P, Crofford O, Davis M, Rand L, Siebert C; Diabetes Control and Complications Trial Research Group. The effect of intensive treatment of diabetes on the development and progression of long-term complications in insulin-dependent diabetes mellitus. N Engl J Med 1993;329:977-86.
48. Nathan DM, Cleary PA, Backlund JY, Genuth SM, Lachin JM, Orchard TJ, Raskin P, Zinman B; Diabetes Control and Complications Trial/Epidemiology of Diabetes Interventions and Complications (DCCT/EDIC) Study Research Group. Intensive diabetes treatment and cardiovascular disease in patients with type 1 diabetes. N Engl J Med 2005;353:2643-53.
49. Cleary PA, Orchard TJ, Genuth S, Wong ND, Detrano R, Backlund JY, Zinman B, Jacobson A, Sun W, Lachin JM, Nathan DM; DCCT/EDIC Research Group. The effect of intensive glycemic treatment on coronary artery calcification in type 1 diabetic participants of the diabetes control and complications trial/epidemiology of diabetes interventions and complications (DCCT/EDIC) study. Diabetes 2006;55:3556-65.
50. Polak JF, Backlund JY, Cleary PA, Harrington AP, O'Leary DH, Lachin JM, Nathan DM; DCCT/EDIC Research Group. Progression of carotid artery intima-media thickness during 12 years in the diabetes control and complications trial/epidemiology of diabetes interventions and complications (DCCT/EDIC) study. Diabetes 2011;60:607-13.
51. UK Prospective Diabetes Study (UKPDS) Group. Intensive blood-glucose control with sulphonylureas or insulin compared with conventional treatment and risk of complications in patients with type 2 diabetes (UKPDS 33). Lancet 1998;352:837-53.
52. Holman RR, Paul SK, Bethel MA, Matthews DR, Neil HA. 10-year follow-up of intensive glucose control in type 2 diabetes. N Engl J Med 2008;359:1577-89.
53. Gerstein HC, Miller ME, Byington RP, Goff DC Jr, Bigger JT, Buse JB, Cushman WC, Genuth S, Ismail-Beigi F, Grimm RH Jr, Probstfield JL, Simons-Morton DG, Friedewald WT; Action to Control Cardiovascular Risk in Diabetes Study Group. Effects of intensive glucose lowering in type 2 diabetes. N Engl J Med 2008;358:2545-59.
54. Advance Collaborative Group, Patel A, MacMahon S, Chalmers J, Neal B, Billot L, Woodward M, Marre M, Cooper M, Glasziou P, Grobbee D, Hamet P, Harrap S, Heller S, Liu L, Mancia G, Mogensen CE, Pan C, Poulter N, Rodgers A, Williams B, Bompoint S, de Galan BE, Joshi R, Travert F. Intensive blood glucose control and vascular outcomes in patients with type 2 diabetes. N Engl J Med 2008;358:2560-72.
55. Duckworth W, Abraira C, Moritz T, Reda D, Emanuele N, Reaven PD, Zieve FJ, Marks J, Davis SN, Hayward R, Warren SR, Goldman S, McCarren M, Vitek ME, Henderson WG, Huang GD; VADT Investigators. Glucose control and vascular complications in veterans with type 2 diabetes. N Engl J Med 2009;360:129-39.
56. Riddle MC. Effects of intensive glucose lowering in the management of patients with type 2 diabetes mellitus in the action to control cardiovascular risk in diabetes (ACCORD) trial. Circulation 2010;122:844-6.
Cite This Article
How to Cite
Download Citation
Export Citation File:
Type of Import
Tips on Downloading Citation
Citation Manager File Format
Type of Import
Direct Import: When the Direct Import option is selected (the default state), a dialogue box will give you the option to Save or Open the downloaded citation data. Choosing Open will either launch your citation manager or give you a choice of applications with which to use the metadata. The Save option saves the file locally for later use.
Indirect Import: When the Indirect Import option is selected, the metadata is displayed and may be copied and pasted as needed.
About This Article
Copyright
Author Biographies
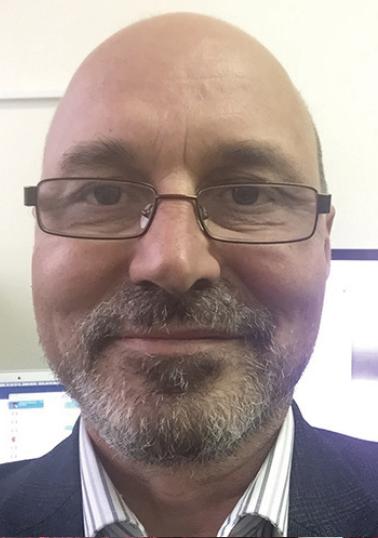
Data & Comments
Data
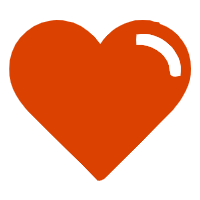
Comments
Comments must be written in English. Spam, offensive content, impersonation, and private information will not be permitted. If any comment is reported and identified as inappropriate content by OAE staff, the comment will be removed without notice. If you have any queries or need any help, please contact us at [email protected].