Essential neural anatomy for creating a clinically translatable osseointegrated neural interface for prosthetic control in sheep
Abstract
Aim: Ovine models for osseointegrated prosthetics research are well established, but do not consider neural control of advanced prostheses. The validity of interfacing technologies, such as the Osseointegrated Neural Interface (ONI), in their ability to provide communication between native nerves and advanced prosthetics is required, necessitating a stable, longitudinal large animal model for testing. The objective of this study is to provide a detailed anatomic description of the major nerves distal to the carpal and tarsal joints, informing the creation of a chronic ONI for prosthetic control in sheep.
Methods: Six pelvic and six thoracic cadaveric limbs from mature female, non-lactating sheep were utilized. Radiographs were obtained to determine average bone length, medullary canal diameter, and cortical bone thickness. Microsurgical dissection was performed to discern topographical neuroanatomy and average circumferences of the major nerves of the pelvic and thoracic limbs. Histologic analysis was performed. A surgical approach for the creation of ONI was designed.
Results: Average metacarpal and metatarsal length was 15.0 cm (± 0.0) and 19.7 cm (± 1.0), respectively. Average intramedullary canal diameter was 12.91 mm (± 3.69) for forelimbs and 12.60 mm (± 3.69) for hindlimbs. The thoracic limb nerves consisted of one dorsal and three ventral nerves, with an average circumference of 5.14 mm
Conclusions: These anatomic data inform the surgical approach and manufacture of a sensory ONI for chronic testing in awake, freely ambulating animals for future clinical translation.
Keywords
INTRODUCTION
The use of large animals as preclinical models is a key step in the translation of biomedical research towards their ultimate application in human patients. Robust, long-term simulation in large animal models is essential to adequately assess the safety and function of any device or intervention prior to human testing. There have been recent technological advances in methods of neural interfacing, such as the Osseointegrated Neural Interface (ONI), which have profound implications for the human amputee population. These interfaces, in combination with advanced prostheses, hold the promise of bi-directional communication in the form of intuitive motor control and sensory feedback between an amputee and their prosthesis; the validity of these new technologies to provide chronic and stable communication between native nerves and advanced prosthetics is required[1-3]. Dingle et al. have demonstrated the durability of ONI in a rabbit model, but furthering this objective requires longitudinal studies in a clinically translatable large animal model[3-4].
Analysis of currently available animal models in neural prosthetic interfacing was recently performed by Aman et al. This article identified that many pilot studies utilize the rat model and rabbit model. Many limitations to these smaller animal models were discussed, including biological differences between rats and humans as well as high infection and self-mutilation rates in rabbit models. Most importantly, though, more comparable anatomy is required to test human-sized devices in a model over time to prove their safety, longevity, and efficacy, which is not possible in small animal models. This article identified a relative dearth of large animal models for the chronic evaluation of peripheral neural interfacing, with no published large animal models for the evaluation of Osseointegrated Neural Interfacing[5].
One ungulate model has been published. Clites et al. demonstrated the utility of a caprine (goat) transtibial and transfemoral hindlimb amputation model for the evaluation of agonist-antagonist myoneural interfacing for prosthetic control. This study demonstrated the chronic stability and safety of an osseointegrated prosthetic device up to day 190 in transfemoral amputation, showing promise for this model in the field of ONI[6]. Similarly, ovine (sheep) models are well established for osseointegrated prosthetics (OI) research[7-10]. Adult sheep share a similar size, weight, and bone structure to adult humans, and thus are an accepted large-animal model for bone and implant biomechanics[11]. The standard ovine model for osseointegration consists of a metacarpal amputation of the thoracic limb as an analogy for transtibial amputation in humans. The ovine model has become the gold standard for long-term large animal OI evaluation, with studies showing stability of at least two years[7]. It follows, then, that the gold standard for ONI using OI prostheses should be this same clinically robust model: the sheep.
Existing OI ovine models do not consider the implications for neural control of the advanced prosthesis. While there are generalized topographical maps of the nerves of the thoracic and pelvic limbs of sheep, more granular information, particularly distal to the carpal and tarsal joints where the amputation is performed, is lacking. The data are crucial for creating a suitable osseointegrated prosthesis with sensory feedback based on the relative sheep models of osseointegration.
The purpose of this study is to elucidate the neural topography in the metatarsi and metacarpi of sheep relative to the common amputation sites for osseointegration research in ungulates. The data obtained from this anatomical study will then be utilized to develop a surgical design for creating an osseointegrated neural interface in a clinically translatable sheep model, alongside similar anatomical studies in humans for direct comparison[12].
METHODS
This work was not conducted with living animals or human subjects. No ethical approval was required.
Animals
Six pelvic and six thoracic cadaveric limbs from mature female, non-lactating sheep (mixed breeds: Polypay, Tarhee, Dorset) were collected for anatomical study. Both forelimbs (thoracic) and hindlimbs (pelvic) were obtained from previous studies, fresh frozen prior to dissection in a standard freezer at -20 ºC. No embalming fluid was used for preservation.
Radiological evaluation of the metacarpal and metatarsal bones
Limbs disarticulated at the carpal and tarsal joints were imaged in pairs using a portable digital radiography system (VetRocket, Santa Clara, CA, USA) including an Elkin EDR3 (Sound, Carlsbad, CA), Canon CXDI-31 plate (Canon USA, Inc., Melville, NY, USA) and a min-X HF100/30+ generator (MinXray, Inc, Northbrook, IL, USA). Each limb was radiographed in the medial-lateral transverse plane alongside a 10 cm radiological scale bar. Measurements of metatarsal and metacarpal bones were made using the straightline tool in ImageJ (NIH, Bethesda, MD), with the scale set according to the scale bar present in each image. Horizontal measurements of the dorsal and palmar cortex bone thickness and medullary canal diameter were made at the proximal, midpoint, and distal positions, as demonstrated in Figure 1. All measurements were made in triplicate and averaged to limit human error. Vertical measurements of bone length were made in the medial plane during microsurgical dissection measuring from the proximal point (carpal/tarsal joint) to the distal point (MP joint), as these anatomic landmarks are more easily identifiable intraoperatively relative to exposed nerves.
Figure 1. Radiological Evaluation of the Metacarpal and Metatarsal Bones. Example radiograph used to obtain skeletal anatomic measurements. Red dashed lines demonstrate the level at which measurements were made, i.e., proximal, midpoint, and distal metacarpi or metatarsi. Blue line indicates intramedullary diameter. Yellow line indicates cortical thickness.
Microsurgical dissection of the nerves distal to the carpal and tarsal joints
Microsurgical dissection was performed by a single author (K.G.) with the assistance of other authors (W.Z., S.O., Z.N.) to determine the overall topography of the major nerves of the pelvic (superficial fibular, deep fibular, and tibial) and thoracic limbs (superficial radial, dorsal ulnar, deep ulnar, and median) along the metatarsi and metacarpi, respectively. The anatomical locations were selected for their relative positioning to the common amputation site for osseointegration models in sheep, measuring and annotating branch points of each nerve to determine viable interfacing targets for creating an ONI. Limbs were dissected using clean, non-sterile microsurgery equipment (Roboz Surgical Instrument Company, MD, USA; Dumont Switzerland, CH) with a Zeiss West Germany Universal Microscope (S3, 175348; NY, USA). Two researchers were present at every dissection to ensure proper measurement recording and nerve identification. Nerve circumferences were measured at three sites along the metatarsus or metacarpus: the most proximal point (at the carpal/tarsal joint), the midpoint (halfway between the carpal/tarsal and interphalangeal (IP) joints), and the most distal point (IP joint). Measurements were made by wrapping a
Morphological examination of the nerves distal to the carpal and tarsal joints
Histological analysis was performed on nerve sections stained with Gomori’s trichrome to identify epineurial thickness and number of fascicles for each nerve as previously reported[3,4,13]. In brief, 1cm samples of each nerve were taken at the proximal, midpoint, and distal positions. Proximal samples were taken 1cm distal to the proximal landmark, the most proximal landmark being the carpal/tarsal joint crease. Distal samples were taken 1cm proximal to the point where the nerve ends or 1cm proximal to the end of the metacarpus or metatarsus. Midpoint samples were taken from the point directly between the most proximal and distal landmarks. All nerve samples were fixed in 10% neutral buffered formalin at 4 °C overnight and processed through graded alcohols in an automated tissue processor (Sakura VIP 5 Tissue Processor, LabX, Ontario, Canada). Processed samples were cut in half transversely, and the proximal and distal transverse surfaces placed face down and paraffin embedded. Transverse serial sections were taken in 5 µM increments, stained with Gomori’s trichrome, and scanned at 20x magnification using a PathScan Enabler IV (Meyer Instruments, Huston, TC, USA). Images were viewed and analyzed using Aperio Image Scope software (v12.3.3.5048, Leica Biosystems, Wetzlar, Germany). Using the freehand selection tool, the entirety of the nerve area was outlined to obtain the total section area (TSA). The number of nerve fascicles was manually counted and the area of each nerve fascicle was measured in the same manner as the TSA. The fascicular area for each nerve was then summed to calculate the total fascicular area (TFA). Average values for TSA, TFA, and number of fascicles were calculated as mean ± standard deviation.
Surgical approach for creation of ONI ovine model
A thoracic cadaveric sheep limb was utilized to test and document the feasibility of the surgical design. The anatomic and histologic data gathered above informed nerve selection and electrode design.
RESULTS
Radiological evaluation of the metacarpal and metatarsal bones
The average intramedullary canal diameter was 12.91 (± 3.69) mm for thoracic limbs and 12.60 (± 3.69) mm for pelvic limbs, with the canal being larger in diameter at the proximal and distal locations than at the midpoints. The average cortical bone thickness was 3.23 (± 0.91) mm in the thoracic limbs and 3.30 (± 0.82) mm in the pelvic limbs [Table 1]. Bone measurements demonstrated an average metacarpal length of 15.0 (± 0.0) cm and an average metatarsal length of 19.7 (± 1.0) cm.
Radiological evaluation of the metacarpal and metatarsal bones
Limb - location | Intramedullary diameter (mm) | Cortical thickness (mm) |
Thoracic - proximal | 14.15 | 4.05 |
Thoracic - midpoint | 8.72 | 3.73 |
Thoracic - distal | 15.86 | 2.52 |
Pelvic - proximal | 15.21 | 2.92 |
Pelvic - midpoint | 10.43 | 3.71 |
Pelvic - Distal | 12.16 | 3.28 |
Microsurgical dissection of the nerves distal to the carpal and tarsal joints
Thoracic limb nerves consisted of one dorsal (superficial radial) and three ventral nerves (dorsal ulnar, deep ulnar, median), with varying branching patterns, with an average circumference of 5.12 (± 1.07) mm dorsally and 4.83 (± 1.74) mm ventrally at the midpoint. Branching patterns of a representative sample of limbs, the right thoracic limbs (n = 3) and right pelvic limbs (n = 3) of each animal are demonstrated in Figure 2. The nerve circumference of each branching nerve at three locations along the metacarpus is demonstrated in Table 2.
Figure 2. Topographical Neural Anatomy. Representative drawings of topographical neural anatomy of the thoracic (A and B) and pelvic (C and D) sheep limbs in dorsal (A and C) and palmar (B and D) planes. Individual branching patterns of n = 3 sheep limbs are demonstrated by different colors.
Neural anatomy results
Nerve | Position | Number of limbs with this nerve (of total 6 limbs) | Average circumference (mm) | Std |
Thoracic limb - dorsal | ||||
Superficial radial nerve | Proximal | 3 | 6.39 | 0.95 |
Medial branch of superficial radial nerve | Proximal | 3 | 5.11 | 0.19 |
Lateral branch of dorsal common digital nerve | Proximal | 3 | 6 | 0.6 |
Medial branch of dorsal common digital nerve | Midpoint | 6 | 5.42 | 1.61 |
Medial branch of dorsal common digital nerve | Distal | 6 | 4.47 | 0.81 |
Central branch of dorsal common digital nerve | Midpoint | 3 | 4.61 | 0.7 |
Central branch of dorsal common digital nerve | Distal | 4 | 4.59 | 1.23 |
Lateral branch of dorsal common digital nerve | Midpoint | 6 | 5.08 | 0.39 |
Lateral branch of dorsal common digital nerve | Distal | 6 | 4.28 | 0.74 |
Thoracic limb - palmar | ||||
Median nerve | Proximal | 6 | 7.25 | 0.88 |
Median nerve | Midpoint | 6 | 7.20 | 0.92 |
Medial branch of median nerve | Distal | 6 | 6.25 | 1.52 |
Lateral branch of median nerve | Distal | 6 | 5.61 | 0.74 |
Communicating branch of median nerve | Distal | 6 | 4.86 | 0.82 |
Deep branch of ulnar nerve | Proximal | 6 | 4.56 | 1.05 |
Deep branch of ulnar nerve | Midpoint | 6 | 3.33 | 0.56 |
Deep branch of ulnar nerve | Distal | 6 | 4.42 | 1.38 |
Dorsal branch of ulnar nerve | Proximal | 6 | 6.28 | 1.56 |
Dorsal branch of ulnar nerve | Midpoint | 2 | 4.42 | 0.12 |
Dorsal branch of ulnar nerve | Distal | 1 | 2.67 | N/A |
Long branch of dorsal ulnar nerve | Distal | 5 | 4.23 | 0.81 |
Long branch of dorsal ulnar nerve | Midpoint | 4 | 3.92 | 1.04 |
Terminating branch of dorsal ulnar nerve | Midpoint | 4 | 4.79 | 1.47 |
Terminating branch of dorsal ulnar nerve | Distal | 4 | 3.78 | 0.95 |
Pelvic limb - dorsal | ||||
Superficial fibular nerve | Proximal | 6 | 8.28 | 1.76 |
Superficial fibular nerve | Midpoint | 6 | 7.45 | 2.1 |
Central branch of dorsal common digital nerve | Distal | 5 | 6.67 | 0.75 |
Central/lateral dorsal common digital nerve | Distal | 1 | 4 | N/A |
Lateral branch of dorsal common digital nerve | Midpoint | 6 | 5.39 | 1.7 |
Lateral branch of dorsal common digital nerve | Distal | 5 | 5.17 | 1.16 |
Medial branch of dorsal common digital nerve | Midpoint | 1 | 6.5 | N/A |
Medial branch of dorsal common digital nerve | Distal | 3 | 6.05 | 1.07 |
Deep fibular nerve | Proximal | 6 | 6.83 | 1.54 |
Central branch of dorsal metatarsal nerve | Midpoint | 6 | 5.92 | 1.45 |
Central branch of dorsal metatarsal nerve | Distal | 6 | 4.92 | 1.32 |
Medial branch of dorsal metatarsal nerve | Midpoint | 1 | 6.33 | N/A |
Medial branch of dorsal metatarsal nerve | Distal | 1 | 4.5 | N/A |
Pelvic limb - plantar | ||||
Tibial nerve | Proximal | 6 | 8.2 | 0.88 |
Lateral plantar nerve | Proximal | 6 | 5.31 | 0.79 |
Lateral plantar nerve | Midpoint | 6 | 5.53 | 0.44 |
Lateral plantar nerve | Distal | 6 | 5.2 | 1.18 |
Medial plantar nerve | Proximal | 6 | 6.42 | 1.33 |
Medial plantar nerve | Midpoint | 6 | 5.28 | 0.62 |
Medial plantar nerve | Distal | 6 | 6 | 0.82 |
On the ventral thoracic limb, the median nerve, deep ulnar nerve, and dorsal ulnar nerve are always present at the proximal point, listed from medial to lateral in their positions in the horizontal plane. The median nerve course is quite consistent. It traversed straight down the ventral metacarpus and reliably branched distal to the midpoint but proximal to the distal point into a medial and lateral branch. Additionally, there is a communicating nerve branch that connects from the median nerve to the deep branch of the ulnar nerve just distal to the midpoint in every animal.
The deep branch of the ulnar nerve began superficially and dove deep beneath a fibrinous sheath to course the length of the metacarpus without branching.
The dorsal branch of the ulnar nerve begins its course on the metacarpus on the ventral side and runs along the lateral edge of the bone, sometimes coursing further onto the dorsal aspect of the limb. The nerve branches into a shorter branch named the terminating branch of the dorsal branch of the ulnar nerve, as well as a longer branch, named the long branch of the dorsal branch of the ulnar nerve, in all but one limb. The branching point was sometimes proximal to the midpoint and other times distal to the midpoint.
On the dorsal thoracic limb, half of the limbs began with a single superficial radial nerve that branched just after the proximal point into a lateral and central branch of the dorsal common digital nerve. Subsequently, one of these then branched once more and traversed distally as the central, medial, and lateral branches of the dorsal common digital nerve. On the other half of the limbs, the superficial radial nerve branched just above the proximal point into the superficial radial nerve proper and the medial branch of the superficial radial nerve. While the superficial radial nerve properly branched as it had in the other limbs into the medial, lateral, and central branches of the dorsal common digital nerves, this medial branch of the superficial radial nerve reliably never branched and continued its course down the metacarpus.
Pelvic limb nerves consisted of two dorsal nerves (superficial and deep fibular) and one ventral (tibial) nerve. Nerves had an average circumference of 6.27 (± 1.79) mm dorsally and 5.40 (± 0.53) mm ventrally at the midpoint. Branching patterns of a representative sample of limbs are demonstrated in Figure 2. The nerve circumference of each branching nerve at three locations along the metatarsus is demonstrated in Table 2.
The ventral pelvic limb had the most consistent neural topography at the level of the metatarsus, with the tibial nerve branching into the lateral plantar and medial plantar nerves. The branching point was always just above the proximal point or within the proximal one-half of the metatarsal.
On the dorsal pelvic limb, the superficial and deep fibular nerves were always present at the proximal point. The superficial fibular nerve branches into the dorsal common digital nerves. Even when it does not branch, by convention, its name is changed to the central branch of the dorsal common digital nerve after the midpoint. The deep fibular nerve branches into the dorsal metatarsal nerves. Even when it does not branch, by convention, its name is changed to the central branch of the dorsal metatarsal nerve at the midpoint. The points of bifurcation of the superficial fibular nerve were widely variable. The deep fibular nerve only bifurcated in one limb. The location of superficial and deep fibular nerves in the horizontal plane at the level of the tarsal joint varied from animal to animal, as demonstrated in Figure 2.
Morphological examination of the nerves distal to the carpal and tarsal joints
The morphological analysis revealed that the average cross-sectional sample contained a rounded average of 10 fascicles within the extracellular matrix. The median number of fascicles was found to be 8 across all samples. The average fascicular area across all samples was 283,108.35 µM2. At 846,645.3 µM2, samples of the lateral plantar nerve taken 1cm proximal of the crease of the ankle had the largest average fascicular area. The proximal and distal samples of this nerve had 16 and 13 fascicles, respectively. On average, the fascicular area constituted 15.23% of the total nerve area. The nerve with the greatest fascicular area was the proximal bifurcation of the lateral plantar nerve, constituting 39.43% of the total nerve area. Detailed results of morphological neural analysis can be found in Table 3. Histological cross-sections of a sample of nerves are demonstrated in Figure 3.
Figure 3. Histological Cross-Sections. Representative micrographs of the nerves of the dorsal aspect of the ovine thoracic limb from a single animal (Proximal to distal). (A) Superficial radial nerve; (B) Lateral branch of the radial nerve; (C) Central branch of the radial nerve; (D and E) Branches of the terminating branch of the dorsal ulnar nerve; (F) Lateral branch of the dorsal common digital nerve; (G) Central branch of the dorsal common digital nerve; (H) Medial branch of the dorsal common digital nerve. Stain: Gomori’s trichrome. Magnification x4, scale bar = 600 µM.
Results of morphological neural analysis
Nerve name | Position | Average nerve area (uM2) | Standard deviation of nerve area (uM2) | Average total fascicular area (uM2) | Standard deviation of total fascicular area (uM2) | Total fascicular area (%) | Total extracellular matrix (%) |
Medial branch radial N. | Proximal | 1,313,621.363 | 412,180.6304 | 116,159.12 | 18,430.87697 | 8.842663742 | 91.15733626 |
Central / lateral branch radial N. | Proximal | 1,892,015.565 | 168,331.4299 | 237,425.4867 | 82,985.04038 | 12.54881255 | 87.45118745 |
Medial branch radial N. | Midpoint | 1,528,413.842 | 287,019.6112 | 80,721.845 | 30,706.83365 | 5.281412847 | 94.71858715 |
Central branch of radial N. | Midpoint | 1,550,498.694 | 951,764.8618 | 431,248.65 | 158,927.0151 | 27.81354488 | 72.18645512 |
Lateral branch of radial N. | Midpoint | 1,533,614.68 | 807,205.5485 | 108,289.55 | 61,751.90331 | 7.061066343 | 92.93893366 |
Medial branch radial N. | Distal | 1,735,489.264 | 447,020.0519 | 79,376.4175 | 47,947.76387 | 4.573719882 | 95.42628012 |
Central branch of radial N. | Distal (3.5 cm distal to midpoint) | 1,072,624.97 | 509,263.4167 | 82,661.6675 | 27,200.23345 | 7.706483609 | 92.29351639 |
Lateral branch of radial N. | Distal | 1,537,271.872 | 436,733.9587 | 79,250.721 | 15,100.21843 | 5.155283359 | 94.84471664 |
Superficial radial N. | Proximal | 2,347,674.918 | 1,193,555.59 | 128,505.46 | 15,423.95929 | 5.473733139 | 94.52626686 |
Branch of terminating branch of dorsal ulnar N. | Midpoint | 839,925.775 | N/A* | 44,004.65 | N/A* | 5.239111754 | 94.76088825 |
Branch of terminating branch of dorsal ulnar N. | Distal | 962,672.64 | N/A* | 64,457.65 | N/A* | 6.695697719 | 93.30430228 |
Central / medial branch of radial N. | Midpoint | 2,066,971.175 | N/A* | 228,208.705 | N/A* | 11.04072992 | 88.95927008 |
Central / lateral branch radial N. | Midpoint | 1,596,757.93 | N/A* | 45,194.945 | N/A* | 2.830419323 | 97.16958068 |
Central / lateral branch radial N. | Distal | 1,303,129.315 | N/A* | 78,874.895 | N/A* | 6.052729694 | 93.94727031 |
Median N. | Proximal | 2,406,305.358 | 1,125,733.976 | 574,786.6892 | 237,869.2077 | 23.8866895 | 76.1133105 |
Median N. | Midpoint | 2,920,078.987 | 1,489,425.397 | 822,654.8525 | 185,020.2374 | 28.17234932 | 71.82765068 |
Median branch median N. | Distal | 1,997,311.363 | 425,958.1426 | 766,990.1033 | 223,089.9885 | 38.40112852 | 61.59887148 |
Lateral branch median N. | Distal | 1,483,023.419 | 746,634.8083 | 183,019.0279 | 98,074.25392 | 12.34093984 | 87.65906016 |
Communicating branch of median N. | Distal | 1,434,057.461 | 128,450.834 | 162,295.6858 | 65,875.57429 | 11.3172373 | 88.6827627 |
Dorsal branch ulnar N. | Proximal | 2,222,570.797 | 785,671.6911 | 190,916.405 | 113,231.3332 | 8.589890828 | 91.41010917 |
Dorsal Branch Ulnar N. | Midpoint | 876,619.125 | 354,876.52 | 242,544.5325 | 183,989.7225 | 27.66817716 | 72.33182284 |
Dorsal branch ulnar N. | Distal | 540,561.21 | N/A* | 35,379.02 | N/A* | 6.544868434 | 93.45513157 |
Terminating branch of dorsal ulnar N. | Midpoint | 1,060,977.388 | 181,045.7686 | 54,620.88625 | 35,579.1525 | 5.148166859 | 94.85183314 |
Terminating branch of dorsal ulnar N. | Distal | 1,560,086.195 | 896,131.7873 | 56,645.945 | 45,989.14068 | 3.630949699 | 96.3690503 |
Long branch of dorsal ulnar N. | Midpoint | 1,377,394.735 | 220,426.1151 | 125,758.3388 | 26,724.30042 | 9.130159681 | 90.86984032 |
Long branch of dorsal ulnar N. | Distal | 1,438,430.212 | 282,639.8785 | 143,960.12 | 123,698.8446 | 10.00814074 | 89.99185926 |
Deep branch ulnar N. | Proximal | 1,141,498.47 | 784,187.291 | 117,954.0983 | 85,557.96491 | 10.33326819 | 89.66673181 |
Deep branch ulnar N. | Midpoint | 1,272,470.884 | 1,024,607.919 | 212,518.6942 | 106,468.3658 | 16.70126184 | 83.29873816 |
Deep branch ulnar N. | Distal | 1,562,244.878 | 803,220.2557 | 278,520.6333 | 89,729.98326 | 17.82823149 | 82.17176851 |
Tibial N. | 1 cm Prox. Of bifurcation of M/L plantar | 2,032,110.05 | 989,502.4044 | 457,189.5675 | 157,432.8918 | 22.49826812 | 77.50173188 |
Medial plantar N. | 1 cm distal. To ankle crease | 2,444,967.505 | 244,404.68 | 474,353.615 | 68,091.135 | 19.4012237 | 80.5987763 |
Lateral plantar N. | 1 cm distal to ankle crease | 3,001,371.913 | 1,675,028.723 | 609,126.86 | 228,210.965 | 20.2949477 | 79.7050523 |
Medial plantar N. | 1 cm Prox. To ankle crease | 1,703,571.425 | 2,257.495 | 464,374.9125 | 22,725.9825 | 27.25890477 | 72.74109523 |
Lateral plantar N. | 1 cm Prox. To ankle crease | 2,884,707.655 | 525,624.94 | 846,645.2875 | 42,027.9875 | 29.34943116 | 70.65056884 |
Medial plantar N. | Distal (at Distal point) | 1,573,032.03 | 86,276.895 | 313,865.8525 | 132,873.8875 | 19.95292191 | 80.04707809 |
Lateral plantar N. | Distal (at Distal point) | 1,757,430.045 | 705,338.245 | 298,924.1175 | 148,620.4825 | 17.00916166 | 82.99083834 |
Lateral plantar N. | Distal (1 cm Prox. To bifurcation of Lat. Plantar) | 1,143,518.22 | N/A* | 450,854.055 | N/A* | 39.4269236 | 60.5730764 |
Medial plantar N. | Distal (1 cm Prox. To bifurcation of Med. Plantar) | 1,150,726.805 | N/A* | 363,439.995 | N/A* | 31.58351691 | 68.41648309 |
Medial plantar N. | Distal (1 cm Prox. To bifurcation of Lat. Plantar) | 1,018,252.33 | N/A* | 357,795.265 | N/A* | 35.13817297 | 64.86182703 |
Lateral plantar N. | Distal (1 cm Prox. To bifurcation of Med. Plantar) | 1,429,746.225 | N/A* | 232,745.025 | N/A* | 16.27876479 | 83.72123521 |
Tibial N. | Proximal | 924,379.7175 | 34,752.3275 | 291,907.675 | 9,556.83 | 31.57876244 | 68.42123756 |
Medial plantar N. | Proximal | 2,282,602 | 591,380.565 | 397,653.075 | 6,225.9 | 17.42104296 | 82.57895704 |
Lateral plantar N. | Proximal | 3,651,584.443 | 296,939.8925 | 597,143.0025 | 145,304.6625 | 16.35298353 | 83.64701647 |
Medial plantar N. | Mid-point | 1,850,289.553 | 359,026.7493 | 187,006.1675 | 47,972.77513 | 10.10685961 | 89.89314039 |
Lateral plantar N. | Mid-point | 1,536,098.163 | 446,599.2818 | 240,943.665 | 117,019.211 | 15.68543409 | 84.31456591 |
Medial plantar N. | Distal | 1,575,482.758 | 162,858.6725 | 324,655.6 | 27,510.885 | 20.60673774 | 79.39326226 |
Lateral plantar N. | Distal | 2,804,900.3 | 573,914.25 | 180,291.08 | 29,036.97 | 6.427717948 | 93.57228205 |
Superficial fibular N. | Proximal | 3,576,311.589 | 1,668,744.949 | 464,885.5117 | 234,806.0552 | 12.99902148 | 87.00097852 |
Superficial fibular N. | Midpoint | 4,077,167.3 | N/A* | 629,677.65 | N/A* | 15.44399834 | 84.55600166 |
Superficial fibular N. – lateral branch | Midpoint | 2,416,342.753 | 1,203,104.367 | 333,369.7033 | 184,930.617 | 13.7964576 | 86.2035424 |
Superficial fibular N. - lateral branch | Distal | 2,085,843.065 | 470,742.1874 | 352,372.4317 | 467,105.6561 | 16.89352558 | 83.10647442 |
Superficial fibular N. - lateral branch | Midpoint | 1,813,696.798 | 202,650.9275 | 262,851.49 | 227,605.52 | 14.49258169 | 85.50741831 |
Superficial fibular N. – lateral branch | Distal | 2,725,055.395 | 820,673.1382 | 332,943.6113 | 96,991.87522 | 12.2178658 | 87.7821342 |
Deep fibular N. | Proximal | 2,252,952.532 | 964,165.2333 | 146,231.585 | 108,294.8549 | 6.49066427 | 93.50933573 |
Deep fibular N. | Midpoint | 622,198.445 | 473,211.6494 | 170,060.975 | 117,375.0952 | 27.33227258 | 72.66772742 |
Deep fibular N. | Distal | 1,883,652.097 | 479,382.9502 | 139,601.88 | 20,926,102.57 | 7.411234815 | 92.58876519 |
Deep fibular N. – lateral branch | Midpoint | 1,224,080.495 | N/A* | 181,893.49 | N/A* | 14.85960202 | 85.14039798 |
Deep fibular N. – lateral branch | Distal | 788,843.18 | N/A* | 159,294.335 | N/A* | 20.19340967 | 79.80659033 |
Dorsal common digital N. – lateral branch | Midpoint | 920,457.27 | 44741.7 | 59,917.8625 | 41,165.3425 | 6.50957567 | 93.49042433 |
Dorsal common digital N. – lateral branch | Distal | 2,933,135.915 | N/A* | 838,514.52 | N/A* | 28.58764627 | 71.41235373 |
Dorsal common digital N. | Midpoint | 4,047,344.705 | N/A* | 0 | N/A* | 0 | 100 |
Dorsal common digital N. | Distal | 1,898,824.595 | N/A* | 256,762.675 | N/A* | 13.52219029 | 86.47780971 |
Superficial fibular N. – central/medial branch | Midpoint | 3,944,359.935 | N/A* | 574,950.025 | N/A* | 14.57651012 | 85.42348988 |
Superficial fibular N. - medial branch | Midpoint | 3,625,162.95 | N/A* | 275,322.86 | N/A* | 7.594771981 | 92.40522802 |
Superficial fibular N. - medial branch | Distal | 2,046,172.032 | 691,991.2229 | 224,397.5167 | 250,036.6623 | 10.96669846 | 89.03330154 |
Deep fibular N. – medial branch | Midpoint | 1,181,432.77 | N/A* | 342,545.06 | N/A* | 28.99403747 | 71.00596253 |
Deep fibular N. – medial branch | Distal | 1,418,896.3 | N/A* | 0 | N/A* | 0 | 100 |
Dorsal common digital N. – medial branch | Midpoint | 3,508,577.213 | 283,054.4475 | 0 | N/A* | 0 | 100 |
Dorsal common digital N. – medial branch | Distal | 2,168,133.66 | N/A* | 234,580.705 | N/A* | 10.81947618 | 89.18052382 |
Surgical approach for creation of ONI ovine model
A cadaveric sheep forelimb was utilized for demonstration. A nine-centimeter lazy-S incision was created over the proximal dorsal metacarpal. Dissection of loose areolar tissue revealed the primary interdigital sensory nerves in this area, representing the branches of the Superficial Radial Nerve. As demonstrated in our anatomic data, there is more variation in branching patterns of some nerves rather than others, as well as more variation in nerve circumference of some nerves over others. It was found that the Central Branch is often the thickest with the least variation; thus, the Central Branch was chosen as our target nerve for creating a reproducible sensory neural interface in sheep. Target nerve was transected distally, three centimeters from the carpal joint. Soft tissue overlying the bone was cleared using a hemostat and elevator. A primary corticotomy was made 20 mm distal to the carpal joint on the medial side of the bone with a handheld electric drill, using a 3/16th inch (4.76 mm) drill bit. A secondary, smaller corticotomy was made with a 5/32nd inch (3.97 mm) drill bit 1cm lateral to the primary corticotomy. Nerve circumference data were used to inform the correct choice of size for the cuff electrodes, as well as drill size for the corticotomy.
A neural interface consisting of three spiral silicone cuffs [Figure 4A-Figure C], two of which contain active electrodes [Figure 4A and Figure 4B] for stimulation and recording, and a third with no electrodes that serve to stabilize the interface [Figure 4C], was created as previously described[14]. Spiral nerve cuff electrodes were used based on availability and applicability, but a range of interfaces are applicable.
Figure 4. Surgical Approach. Left: Neural interface. Electrode A is an active electrode capable of delivering sensory stimulation signals. Electrode B is an active electrode, which detects sensory stimulation delivered by Electrode A. Electrode C is a stabilizing electrode. Ruler in image 1 measures millimeters. Right: Image of surgical dissection, as described in text. Electrode A is intramedullary in this photo. Electrode C is not included in this surgical demonstration. Ruler in image 2 measures centimeters.
Electrode A was attached to the distal end of the transected target nerve. Electrode B was then connected more proximally to the nerve, at a point of minimal tension. The target nerve, concurrently with Electrode A, was then transposed into the medullary canal through the primary corticotomy by threading an epineurial propene suture into the primary corticotomy and out the secondary corticotomy and secured to the periosteum. Electrode B remained outside the medullary canal, maintaining the separation of 10cm necessary for stimulation and recording of compound nerve action potentials between electrodes. The distal electrode (A) can then deliver sensory stimulation to be detected by the proximal electrode (B) in situ. The electrode cables then exit transcutaneously and are anchored with suture.
DISCUSSION
The Osseointegrated Neural Interface is a developing technology with an overarching goal of creating a bidirectional prosthesis capable of both motor control and sensory realization[12]. This technology has demonstrated success in a small animal model involving rabbits, but longitudinal studies in a large animal model are necessary before translation to human use can be pursued[3,4]. Building on current clinically translatable ovine models for osseointegration, we studied the topography of ovine nerves distal to the carpal and tarsal joints in order to design and create an ONI suitable for chronic testing in sheep. The data herein were consistent with models of ovine OI[7,8,15].
Bone measurements demonstrate an average metacarpal length of 15.0 (± 0.0) cm and an average metatarsal length of 19.7 (± 1.0) cm. The average cortical bone thickness was 3.23 (± 0.91) mm in the thoracic limbs and 3.30 (± 0.82) mm in the pelvic limbs. The average intramedullary canal diameter was 12.91 (± 3.69) mm for thoracic limbs and 12.60 (± 3.69) mm for pelvic limbs. The thoracic limbs consisted of one dorsal (superficial radial) and three ventral (median, dorsal ulnar, and deep ulnar) nerves, with an average circumference of 5.12 (± 1.07) mm and 4.83 (± 1.74) mm at the midpoint, respectively. Pelvic limb nerves consisted of two dorsal (superficial fibular and deep fibular) and one ventral (tibial) nerve with an average circumference of 6.27 (± 1.79) mm and 5.40 (± 0.53) mm at the midpoint, respectively.
At the level of amputation in the classic ovine OI, the distal metacarpus, all nerves provide cutaneous sensory innervation, with no identifiable motor targets, and thus are the ideal target nerves for the creation of osseointegrated prosthesis with sensory feedback. Nerves were adequate in both length and circumference to support the creation of a neural interface with subsequent transposition into the medullary canal.
Sheep transmetatarsal amputation is the gold standard in OI research, representative of human transtibial amputation. The forelimb anatomy and joint loading are more similar to the human knee joint than that of the hindlimb, which is remarkably complex. The standing orientation of the forelimb is more vertical than that of the hindlimb and provides more even loading of the OI implant[16,8,10,17]. Further, equines and ovines have a unique stay apparatus in their hindlimbs, which negates the use of the hindlimb as a load-bearing model for prostheses. This apparatus is a delicate balance of tendons, ligaments, and muscles that both passively and actively allow them to remain upright with minimal energy consumption[18,19]. If these are transected in the hindlimb, as is the case during amputation, the animal will refuse to bear weight on the limb entirely.
Caprines (goats) do not have such a stay mechanism, which makes a hindlimb model possible, as
There are several different types of OI prostheses – Osseointegrated Prosthesis for the Rehabilitation of Amputees (OPRA), a screw-type prosthesis, Osseointegrated Prosthetic Limb (OPL), and the Integral Leg Prosthesis (ILP), both of which are press-fit prostheses. The proposed model for ONI testing in sheep would be compatible with all of these devices. Importantly, in the surgical approach delineated in this article, it is noted that wires are passed subcutaneously and out of the skin to connect with a recording and stimulating device. In the final design of the osseointegrated neural interface, the communicating wires travel through the osseointegrated implant and communicate with the prosthesis through the osseointegrated abutment[12]. The e-OPRA implant is a modification of the OPRA implant designed specifically to allow bi-directional interfacing via the medullary canal. Given that the sheep model for OI is well established, the focus of this article was the relevant neural anatomy and surgical approach required to construct the neural interfacing componentry of an ONI.
There are limitations to this study. This study was performed on cadaveric sheep limbs and thus anatomy, specifically nerve circumference, may not be fully representative of the awake and ambulating sheep. Importantly, sheep were fresh frozen and not preserved in formalin or other alcohol in order to preserve anatomy to the highest possible degree. Additionally, the low number of specimens is a limitation. There may be anatomical variations that were not picked up, given the low number of sheep limbs dissected. The study herein provides valuable granular information in two dimensions in space, the proximal/distal and the medial/lateral dimension. However, this study is limited in its ability to provide further data in the anterior to posterior dimension to the audience. Importantly, this dimension was considered during dissection when determining easy and appropriate target nerves for our surgical approach, but granular data were not collected to provide in text.
CONCLUSIONS
The present study provides essential anatomic data for creating an ovine osseointegrated prosthesis with sensory feedback, based on the accepted gold standard sheep model of osseointegration. These data inform the manufacture of a sensory ONI for chronic testing in awake, freely ambulating large animals for future clinical translation.
DECLARATIONS
AcknowledgmentsWe would like to acknowledge the University of Wisconsin Surgery Histology Core and the University of Wisconsin Translational Research Initiatives in Pathology group for their assistance with histology slide creation and image analysis.
Authors’ contributionsConcept, design, data collection/analysis, manuscript drafting: Gunderson KA, Dingle AM
Concept, design, data collection/analysis, manuscript revision: Odorico SK, Nkana ZH, Zeng W
Data collection/analysis, manuscript revision: Sears L, Seils G
Concept, design, figure creation, manuscript revision: Minor RL
Concept, design, data analysis, manuscript revision: Poore SO
Availability of data and materialsData and materials from this study will be provided upon request.
Financial support and sponsorshipNone.
Conflicts of interestAll authors declared that there are no conflicts of interest.
Ethical approval and consent to participateIn accordance with the University of Wisconsin-Madison Institutional Animal. Care and Use Committee’s ethics policies, the ethical review was not required to undertake this research.
Consent for publicationNot applicable.
Copyright© The Author(s) 2023.
REFERENCES
1. Millevolte AXT, Dingle AM, Ness JP, et al. Improving the selectivity of an osseointegrated neural interface: proof of concept for housing sieve electrode arrays in the medullary canal of long bones. Front Neurosci 2021;15:613844.
2. Karczewski AM, Dingle AM, Poore SO. The need to work arm in arm: calling for collaboration in delivering neuroprosthetic limb replacements. Front Neurorobot 2021;15:711028.
3. Dingle AM, Ness JP, Novello J, et al. Methodology for creating a chronic osseointegrated neural interface for prosthetic control in rabbits. J Neurosci Methods 2020;331:108504.
4. Dingle AM, Ness JP, Novello J, et al. Experimental basis for creating an osseointegrated neural interface for prosthetic control: a pilot study in rabbits. Mil Med 2020;185:462-9.
5. Aman M, Bergmeister KD, Festin C, et al. Experimental testing of bionic peripheral nerve and muscle interfaces: animal model considerations. Front Neurosci 2020;13:1422.
6. Clites TR, Carty MJ, Srinivasan SS, Talbot SG, Brånemark R, Herr HM. Caprine models of the agonist-antagonist myoneural interface implemented at the above- and below-knee amputation levels. Plast Reconstr Surg 2019;144:p 218e-229e.
7. Jeyapalina S, Beck JP, Agarwal J, Bachus KN. A 24-month evaluation of a percutaneous osseointegrated limb-skin interface in an ovine amputation model. J Mater Sci Mater Med 2017;28:179.
8. Jeyapalina S, Beck JP, Drew A, Bloebaum RD, Bachus KN. Variation in bone response to the placement of percutaneous osseointegrated endoprostheses: a 24-month follow-up in sheep. PLoS One 2019;14:e0221850.
9. Hoellwarth JS, Tetsworth K, Rozbruch SR, Handal MB, Coughlan A, Al Muderis M. Osseointegration for amputees: current implants, techniques, and future directions. JBJS Rev 2020;8:e0043.
10. Shelton TJ, Beck JP, Bloebaum RD, Bachus KN. Percutaneous osseointegrated prostheses for amputees: limb compensation in a 12-month ovine model. J Biomech 2011;44:2601-6.
11. Sartoretto SC, Uzeda MJ, Miguel FB, Nascimento JR, Ascoli F, Calasans-Maia MD. Sheep as an experimental model for biomaterial implant evaluation. Acta Ortop Bras 2016;24:262-6.
12. Karczewski AM, Zeng W, Stratchko LM, Bachus KN, Poore SO, Dingle AM. Clinical basis for creating an osseointegrated neural interface. Front Neurosci 2022;16:828593.
13. Settell ML, Pelot NA, Knudsen BE, et al. Functional vagotopy in the cervical vagus nerve of the domestic pig: implications for the study of vagus nerve stimulation. J Neural Eng 2020;17:026022.
14. Blanz SL, Musselman ED, Settell ML, et al. Spatially selective stimulation of the pig vagus nerve to modulate target effect versus side effect. J Neural Eng 2023;20:016051.
15. Jeyapalina S, Beck JP, Bachus KN, Chalayon O, Bloebaum RD. Radiographic evaluation of bone adaptation adjacent to percutaneous osseointegrated prostheses in a sheep model. Clin Orthop Relat Res 2014;472:2966-77.
16. Duda GN, Eckert-Hübner K, Sokiranski R, Kreutner A, Miller R, Claes L. Analysis of inter-fragmentary movement as a function of musculoskeletal loading conditions in sheep. J Biomech 1998;31:201-10.
17. Grisez BT, Hanselman AE, Boukhemis KW, Lalli TAJ, Lindsey BA. Osseointegrated transcutaneous device for amputees: a pilot large animal model. Adv Orthop 2018;2018:4625967.
18. Schuurman SO, Kersten W, Weijs WA. The equine hind limb is actively stabilized during standing. J Anat 2003;202:355-62.
Cite This Article
Export citation file: BibTeX | RIS
OAE Style
Gunderson KA, Odorico SK, Nkana ZH, Sears L, Seils G, Minor RL, Zeng W, Poore SO, Dingle AM. Essential neural anatomy for creating a clinically translatable osseointegrated neural interface for prosthetic control in sheep. Plast Aesthet Res 2023;10:50. http://dx.doi.org/10.20517/2347-9264.2023.42
AMA Style
Gunderson KA, Odorico SK, Nkana ZH, Sears L, Seils G, Minor RL, Zeng W, Poore SO, Dingle AM. Essential neural anatomy for creating a clinically translatable osseointegrated neural interface for prosthetic control in sheep. Plastic and Aesthetic Research. 2023; 10: 50. http://dx.doi.org/10.20517/2347-9264.2023.42
Chicago/Turabian Style
Gunderson, Kirsten A., Scott K. Odorico, Zeeda H. Nkana, Lucas Sears, Grant Seils, Rashea L. Minor, Weifeng Zeng, Samuel O. Poore, Aaron M. Dingle. 2023. "Essential neural anatomy for creating a clinically translatable osseointegrated neural interface for prosthetic control in sheep" Plastic and Aesthetic Research. 10: 50. http://dx.doi.org/10.20517/2347-9264.2023.42
ACS Style
Gunderson, KA.; Odorico SK.; Nkana ZH.; Sears L.; Seils G.; Minor RL.; Zeng W.; Poore SO.; Dingle AM. Essential neural anatomy for creating a clinically translatable osseointegrated neural interface for prosthetic control in sheep. Plast. Aesthet. Res. 2023, 10, 50. http://dx.doi.org/10.20517/2347-9264.2023.42
About This Article
Special Issue
Copyright
Data & Comments
Data
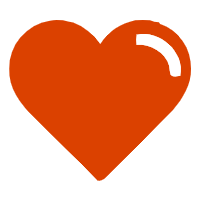

Comments
Comments must be written in English. Spam, offensive content, impersonation, and private information will not be permitted. If any comment is reported and identified as inappropriate content by OAE staff, the comment will be removed without notice. If you have any queries or need any help, please contact us at support@oaepublish.com.