Regenerative peripheral nerve interfaces (RPNIs): an overview of innovative surgical approaches
Abstract
Complications following amputation can be devastating for patients, including debilitating neuropathic pain, the inability to perform activities of daily living (ADLs) or gain meaningful employment. While prosthesis use allows patients to restore independence and reintegrate into daily activities, patients often abandon these devices. Despite the immense advancements in prosthetic technology, there is still a need for an interface that can provide a natural experience with accurate and reliable long-term control. The Regenerative Peripheral Nerve Interface (RPNI) is a simple surgical technique that offers real-time control of myoelectric prosthetic devices to restore extremity function. This stable, biological nerve interface successfully amplifies efferent motor action potentials, provides sensory feedback, and offers a more functional prosthetic device experience. Based on the principles of RPNI, novel surgical approaches have been developed to expand its applications and improve outcomes. This review article summarizes the utilization of the RPNI and its recent modifications of different neural interfaces in the setting of major limb amputation and musculoskeletal injuries.
Keywords
INTRODUCTION
Over 150,000 individuals undergo major limb amputations in the United States annually[1,2]. Up to 80% of these patients experience complications including severe neuropathic pain and limited participation in physical and occupational activities[3]. Unfortunately, this often results in psychological stress and poor quality of life[4]. Prosthesis use allows patients to restore independence and reintegrate into social and occupational activities. Myoelectric prostheses demonstrate advanced capabilities compared with body-powered prosthetic limbs, such as precise hand, wrist, and finger movements and greater degrees of freedom[5]. However, patients abandon these prosthetic devices at varying rates (0%-75%), due to the lack of comfort of the socket, neuroma pain, and the lack of realistic sensory feedback[6,7]. Current prosthetic technologies mostly acquire discrete control signals from the limited number of available sites through surface electrodes, which limits signal fidelity, reliability, and multifunctionality[8]. In addition, the lack of meaningful sensory and proprioceptive feedback, the necessity for frequent recalibration, and the amount of training result in dissatisfaction with these devices[8,9]. Thus, despite the immense developments in prosthetics technology, there is still a need for an interface that can provide a natural experience with optimal activity and control. Allowing a patient to intuitively control more than a single movement at a time is ideal, but this requires separate and independent motor and sensory signals captured from the residual peripheral nerves[8].
Rehabilitation for individuals with amputations is often limited by postamputation pain, including symptomatic neuromas and phantom limb pain (PLP)[10]. This often results in debilitating chronic neuropathic pain that significantly limits prosthetic use and interferes with activities of daily living (ADLs)[11,12]. Current therapeutic interventions are often inadequate, as there is no identified clinical “gold standard” for the surgical treatment of neuromas[13,14]. Therefore, there is a need to develop a robust and reliable technique to address postamputation pain.
The Regenerative Peripheral Nerve Interface (RPNI) has been developed to overcome these problems. The RPNI is a simple and safe technique that serves as a stable, biological nerve interface[15-18]. By leveraging the physiologic processes of nerve regeneration, muscle regeneration, and reinnervation, RPNIs have a dual purpose of providing enhanced motor control of prosthetic devices and reducing postamputation pain[18]. It can be performed after neuroma excision or prophylactically at the time of amputation[19]. Building from experience with the initial RPNI construct, the Dermal Sensory Regenerative Peripheral Nerve Interface (DS-RPNI), Composite Regenerative Peripheral Nerve Interface (C-RPNI), and the Muscle Cuff Regenerative Peripheral Nerve Interface (MC-RPNI) have also been designed. This article reviews current concepts of RPNIs and focuses on the multiple applications of its novel modifications to enhance the functionality of myoelectric prostheses and exoskeleton devices.
REGENERATIVE PERIPHERAL NERVE INTERFACE (RPNI)
The RPNI is a biological interface composed of a transected peripheral nerve implanted into an autologous free skeletal muscle graft[15,16,18]. Initially, all transected peripheral nerves in the residual limb are identified, isolated, and dissected from the surrounding soft tissues[18]. Dissection of large proximal nerves, such as the sciatic nerve, into three or four fascicles is recommended to improve axon-to-muscle fiber ratio[18,20]. For each of the isolated nerves, healthy muscle grafts are harvested parallel to the muscle fibers either directly from the amputated limb or at a distal site with a separate incision[18,20]. The most commonly used graft size to optimize revascularization is 30 to 40 mm long, 15 to 20 mm wide, and 5 to 6 mm thick[18,20]. Finally, transected peripheral nerves are implanted into the center of the muscle graft [Figure 1]. RPNIs should be created in a proximal area, remote from the surgical incision site and weight-bearing surfaces of the residual limb. The time required to implant a single RPNI construct is 7 to 10 min[20].
Figure 1. The Regenerative Peripheral Nerve Interface (RPNI) construct. (A) Intraoperative dissection and isolation of median, ulnar, and radial nerves (B) Implantation of six RPNIs, two each for the median nerve, ulnar nerve, and radial nerve (Burke KL, Kung TA, Hooper RC, Kemp SWP, Cederna PS. Regenerative peripheral nerve interfaces (RPNIs): current status and future direction[8].
Following the procedure, denervated muscle fibers undergo robust reinnervation by regenerating axons, revascularization, and eventually mature RPNI formation[18]. The transition process to a prosthetic limb is supported by training, which involves the control of a real physical or virtual avatar prosthetic limb. Efforts to contract the muscles in the missing limb generate neural signals that allow prosthetic control of corresponding movements. However, recording signals directly from peripheral nerves is challenging because nerve signal amplitudes are small and produce low signal-to-noise ratios (SNRs). Each RPNI has the ability to serve as a biological amplifier without causing iatrogenic nerve injury while providing high amplitude and reliable signals with high SNRs[15,21]. In a rat model, RPNIs with an implanted electrode remained viable over 7 months and the reinnervated muscle graft transduced high-amplitude compound muscle action potentials (CMAPs) from low-amplitude efferent motor action potentials[17]. Further studies in both rodents and non-human primates confirmed the biological stability of the interface and demonstrated successful axonal regeneration and reinnervation by providing histologic evidence[21-24]. In a first-in-human pilot study, four participants with upper extremity amputations underwent RPNI surgery[22]. Two individuals with distal transradial amputations elected to undergo surgical implantation of indwelling intramuscular bipolar electrodes into their RPNIs, 3 years and 12 months after the initial RPNI surgery. The SNRs measured in RPNIs of two patients had mean values of 68.9 and 21.0 decibels (dB)[22]. The variation may be due to the difference in duration between RPNI surgery and electrode implantation since additional years may prolong the period of RPNI maturation and reinnervation[22]. In a follow-up clinical study, researchers further investigated the longevity of the RPNI by assessing the signal reliability from chronically implanted RPNIs[24]. RPNIs with indwelling bipolar electrodes produced SNRs that remained consistently high, ranging from 15 to 250 dB across sessions for up to 276 days and 1,054 days, respectively[24]. The difference in SNR magnitude between sessions was likely due to the variability of the electromyography (EMG) signals rather than shifts in electrode noise[24]. Despite the variation, both SNRs remained significantly higher than the SNR range from 2 to 20 typically recorded from surface EMG, intraneural probes, and nerve cuff electrodes[22,24]. Additionally, by obtaining motor control signals from RPNI, both participants completed functional and real-world tasks (e.g., coffee task, box, and block task) that require various arm positions and grip movements over a period of 16 months without a need for recalibration[24]. This study further showed the potential of RPNIs as a long-term interface for enhanced control of prosthetic devices. By providing individuals with a more intuitive experience and improving quality of life, RPNIs hold promise for significantly advancing prosthetic technology in the future. The high adaptability of this simple and effective surgery has subsequently led to the development of multiple modifications and expanded its applications to different clinical settings, such as musculoskeletal injuries and advanced control of exoskeleton devices.
RPNIs are also an effective surgical intervention in both the prevention and treatment of postamputation pain resulting from symptomatic neuroma[8,16,18,19]. Following amputation, regenerating peripheral nerves without a distal target can cause disorganized bulbous axonal sprouting, leading to subsequent neuroma formation[12,16]. These neuromas may cause spontaneous ectopic neural activity, resulting in hyperalgesia, allodynia, and eventually, chronic neuropathic pain[11,12]. By providing a distal target for regenerating axons, RPNIs may prevent the formation of symptomatic neuromas. In a pilot clinical study, sixteen patients with amputations underwent RPNI surgery for symptomatic postamputation neuromas[25]. Using the numeric pain rating scale, an average reduction of neuroma pain score by 71% and phantom limb pain score by 53% was reported postoperatively. In addition, 75% of patients were satisfied with RPNI surgery and 94% would choose to undergo surgery again when asked on the questionnaire[25]. When RPNIs were implanted prophylactically at the time of amputation, the results indicated a significantly lower incidence of both neuroma and phantom limb pain compared to the control cohort[19]. In this study, postoperative outcomes were evaluated in a total of 90 patients. Forty-five patients underwent RPNI implantation at the time of primary amputation, and 45 control patients underwent amputation without RPNI. Six control patients (13.3 percent) developed symptomatic neuromas in the postoperative period compared with zero (0.0 percent) in the prophylactic RPNI group (P = 0.026). Twenty-three RPNI patients (51.1 percent) reported phantom limb pain, compared with 41 control patients (91.1 percent).
DERMAL SENSORY REGENERATIVE PERIPHERAL NERVE INTERFACE (DS-RPNI)
Sensory feedback is of great importance for efficient motor planning, execution, and accomplishing daily tasks[26]. A prosthesis that lacks sensory and positional feedback is often experienced as foreign to individuals with amputations and may result in user dissatisfaction and abandonment of these devices[26]. Providing a prosthetic device that can restore sensory information to individuals with amputations is, therefore, a critical need. However, currently available prosthetic devices cannot accurately translate sensor-derived information into meaningful, somatotopically matched sensory feedback[9,27]. This was supported by a survey in which prosthetic users reported sensory feedback as one of the main features missing in current devices[27]. Similarly, absence of physiologic feedback is seen in the generation of phantom limb pain. PLP is often burdensome to patients, resulting in displeasure among prosthetic users and subsequent rejection of their prosthetic device[26,27]. Although the mechanism is still unclear, studies have shown improvements in PLP when provided with sensory stimulation[26,27]. Therefore, reestablishing sensory feedback could significantly reduce PLP and relieve the burden of individuals with amputation.
To overcome these limitations, the RPNI has been modified to develop the Dermal Sensory Regenerative Peripheral Nerve Interface (DS-RPNI). The DS-RPNI consists of a residual sensory nerve implanted into a deepithelialized skin graft measuring 0.5 × 1.0 cm [Figure 2][9]. The construct is placed subcutaneously, and the graft is reinnervated by the sensory afferents in two months. A single DS-RPNI can be created for each sensory nerve. To allow for sensory feedback from various distinct locations, multiple sensory nerves can be implanted into independent deepithelialized skin grafts[9]. So far, results from the preclinical study in rodents showed robust revascularization and viable free dermal flap two months after fabrication.
Figure 2. The Dermal Sensory Regenerative Peripheral Nerve Interface (DS-RPNI) construct. (A) Illustrative schematic consisting of deepithelialized skin graft secured around the sensory nerve; (B) DS-RPNI in vivo in a rat at the time of fabrication.
In addition to its potential role of restoring natural sensation in individuals with amputations, DS-RPNI is used in the management of chronic neuropathic pain localized to a specific sensory nerve as an alternative treatment strategy to traditional neurectomy. Hart and Brown reported two cases, one ankle injury and the other mastectomy, to highlight the results of DS-RPNI surgery in patients with chronic pain and positive Tinel’s sign[28]. Visual analog scale pain scores significantly decreased from 9-10 to 1-3, postoperatively[28].
COMPOSITE REGENERATIVE PERIPHERAL NERVE INTERFACE (C-RPNI)
An ideal prosthetic neural interface would be capable of achieving bidirectional motor control and sensory feedback[29]. Thus, RPNI and DS-RPNI were combined to create the novel construct, the Composite Regenerative Peripheral Nerve Interface (C-RPNI). Utilization of both components of this interface simultaneously has the potential to pave the way for the realization of the ideal prosthetic device control strategy.
The C-RPNI is composed of a mixed sensorimotor nerve that is implanted into a construct of a free muscle graft secured to a dermal graft[30]. The central tendinous tissues and a small segment of epimysium are removed from the muscle graft. Following the preparation of dermal and muscle grafts and isolation of the transected nerve, the epineurium of the transected nerve end is secured to the area of the muscle graft where the epimysium was removed. Next, the dermal graft is positioned on the muscle graft where it fully covers the nerve and majority of the muscle. Then, proximal and distal parts of the construct are attached to the femur periosteum to secure the construct in its place [Figure 3][30].
Figure 3. The Composite Regenerative Peripheral Nerve Interface (C-RPNI). (A) Illustrative schematic of the C-RPNI. The peripheral nerve is secured between the top dermal graft and the bottom muscle graft; (B) C-RPNI in vivo immediately following fabrication in a rat. Muscle is marked by (M), dermis (D), and common peroneal nerve (N). (Svientek SR, Ursu DC, Cederna PS, Kemp SWP. Fabrication of the Composite Regenerative Peripheral Nerve Interface (C-RPNI) in the Adult Rat[30].
Peripheral nerves show a preference for the type of end organ they reinnervate[30]. Regenerating motor neurons have a tendency to reinnervate motor pathways, while sensory neurons preferentially innervate cutaneous branches of the nerve[30,31]. The C-RPNI relies on the inherent preferential reinnervation principle of the target nerve itself to facilitate reinnervation. Motor fibers innervate the muscle graft, while the sensory fibers innervate the dermal component of the C-RPNI[30]. In more distal extremity amputations, individual RPNI and DS-RPNI constructs are implanted, since motor and sensory fascicles can be surgically separated. However, at proximal levels, bundles of motor and sensory axons travel together. Thus, surgical separation of the intermingled axons is often difficult and does not allow for separate RPNI and DS-RPNI constructions. Therefore, the C-RPNI would be the preferred RPNI strategy in treating patients who present with proximal, transhumeral amputations[30].
MUSCLE CUFF REGENERATIVE PERIPHERAL NERVE INTERFACE (MC-RPNI)
Approximately 130 million people in the United States are affected by musculoskeletal injuries[32,33]. Musculoskeletal injuries comprise diverse pathologies affecting nerves, neuromuscular junctions, or muscle itself. In contrast to individuals with amputations, these patients have peripheral nerves in continuity and often present with debilitating motor extremity impairment (e.g., brachial plexus injuries, compartment syndrome, volumetric muscle loss). Treatment strategies mostly remain supportive and necessitate usage of mobility aids (e.g., canes, wheelchairs)[32,33]. Recent advances in technology have led to the development of novel wearable exoskeleton devices[34]. The operation principle of these devices is to detect signals from surface electromyography (sEMG) of distal limb muscles or electroencephalography (EEG)[32,33]. However, both signaling pathways have significant drawbacks that limit the utilization of these devices. For example, accurate extraction of neural control signals with EEG can be significantly affected by the location of electrode placement, body temperature, and the presence of sweat and/or hair[32,33,35]. Widespread utilization of both methods is also limited due to reliance on precise sensor positioning, implementation of complex processing algorithms, the necessity for intensive user training, and the need for continual recalibration[35-37]. Transcranial recordings from EEG produce low SNRs and inaccurate signal detection[32,33,35]. Although sEMG signals can overcome these limitations by providing larger amplitude and greater motor detection, signals are prone to crosstalk from deep or adjacent muscles[38]. In addition, when there is a significant pathological muscle compromise, sEMG cannot be used to detect the signals[32,33]. Thus, a novel peripheral nerve interface that provides high-accuracy detection of motor intent to control exoskeleton devices would benefit these patients.
As RPNI was developed for individuals with amputations who have transected peripheral nerves, the construct was modified for peripheral nerves in continuity by developing the Muscle Cuff Regenerative Peripheral Nerve Interface (MC-RPNI)[33]. The MC-RPNI also consists of a free skeletal muscle graft as in the RPNI, but instead, it is secured around a peripheral nerve in continuity [Figure 4]. Extensor digitorum longus (EDL) muscle and the common peroneal (CP) nerve are used in animal models to create the construct. Following the muscle harvest and nerve isolation, the graft is positioned centrally underneath the nerve, approximately 2 cm distal to the sciatic notch[33]. An epineural window is created to allow collateral reinnervation by removing 25% of the epineurium along the anterior surface of the nerve within the MC-RPNI[33]. Then, the muscle graft is wrapped circumferentially around the nerve in continuity. Regeneration and reinnervation through collateral axonal sprouting occur over a three-month maturation period, and the construct can produce dramatically amplified efferent motor action potentials from the nerve[32,33]. The CP nerve branch to the tibialis anterior (TA) muscle or the tendons or muscle belly of the TA is cut away from the EDL, immediately preceding the endpoint EMG recording. As previously shown in the RPNIs, simultaneous control of multiple degrees of freedom can be achieved with the MC-RPNI, as distally dissected nerves can be implanted into individual muscles without significant signal contamination[32,33]. Given its biogenic origin and strategic placement, the MC-RPNI avoids detriment to distally innervated targets, and preclinical rodent studies have demonstrated the lack of downstream muscle compromise[32,33]. Thus, the MC-RPNI has substantial promise for the widespread utilization of safe, accurate, and reliable exoskeleton devices in those living with extremity weakness.
Figure 4. The Muscle Cuff Regenerative Peripheral Nerve Interface (MC-RPNI). (A) Illustrative schematic of the MC-RPNI. The peripheral nerve in continuity innervating its distal target can be seen in yellow within the surrounding muscle graft; (B) MC-RPNI in vivo immediately following fabrication in a rat. Muscle tissue (M) and common peroneal nerve (N).
DISCUSSION
Innovative approaches to human-prosthetic interfacing are continuously advancing neural interface technology and enabling the development of next-generation sophisticated prosthetic limbs. In this review, we provided a detailed description of RPNI technology that takes advantage of the basic muscle and nerve physiology for real-time control of myoelectric prostheses. One of the other promising current approaches to provide intuitive control of advanced myoelectric prostheses is Targeted Muscle Reinnervation (TMR). TMR is a surgical technique developed in the early 2000s for individuals with amputations[39]. In this procedure, residual mixed peripheral nerves from the amputated limb are transferred to small intact motor nerves[39]. Distinct from RPNI, TMR relies on surgical denervation of functional muscle targets so that the newly transferred nerve can reinnervate successfully[39,40]. Following reinnervation, neural signals from transferred nerves cause target muscles to contract, which provides additional EMG signal sites for prosthetic control. TMR has been performed in more than 100 patients at various amputation levels to provide improved degrees of prosthetic control[41]. However, barriers remain to getting intuitive control of the prosthetic limb and more widespread implementation of these surgical techniques. Skin electrodes used in TMR to record sEMG signals demonstrate limitations that impede the precise measurement of muscle contractions[40,42]. Recording electrical activity from the body surface may alter the signal characteristics due to positional and physiological changes[40,42]. In addition, sEMG recordings are prone to crosstalk and interference from adjacent muscles (or other electrodes), which necessitates advanced signal processing systems for the neural interface to be effective[43]. Furthermore, reinnervation of the target muscle by multiple large peripheral nerves results in a limited number of EMG recording sites and overlapping signals, which requires the implementation of complex pattern recognition algorithms to assign the signals to the intended control targets[40]. Thus, TMR demands more technical and scientific proficiency compared to RPNI. In addition to its use as a mechanoneural interface for intuitive prosthetic control, patients may also experience attenuated residual limb pain (RLP) and PLP following TMR[41]. Mioton et al. showed significant reductions in RLP (from 6.4 ± 2.6 to 3.6 ± 2.2) and PLP (from 6.0 ± 3.1 to 3.6 ± 2.9) following TMR surgery[44]. Dumanian et al. conducted the first randomized clinical trial and assigned patients with chronic pain to TMR or standard care with traditional neurectomy[45]. Although they reported a greater improvement in PLP and RLP in the TMR cohort, pain score reduction failed to reach statistical significance[45].
RPNIs are highly effective for the treatment and prevention of neuroma pain and PLP. They are also effective in amplifying peripheral nerve action potentials to facilitate the recording of high-fidelity motor control signals. To date, more than 200 patients with upper and lower extremity amputations underwent RPNI surgery[41]. However, they do not work in all cases, largely due to the need for revascularization of the RPNI during the healing process. Anything that could potentially adversely impact the revascularization of the RPNI may impact the full regeneration and reinnervation of the construct. Although no direct evidence exists, relative contraindications for other soft tissue grafts, such as vascular disease, nutritional deficiency, corticosteroid use, and immunosuppression, may also interfere with the RPNI maturation and revascularization process[46]. For this reason, RPNI is not performed on patients who are actively using nicotine because this will adversely impact the revascularization and regeneration of the RPNI[47,48]. When RPNI is performed in a poorly vascularized bed, such as a significantly scarred or radiated field, full regeneration or reinnervation may also not be achieved due to compromised revascularization[49]. Lastly, it is not recommended to perform RPNIs in contaminated fields, as this may also impact the viability of the RPNI over time.
CONCLUSION
An ideal prosthetic interface would provide bidirectional motor control and sensory feedback. RPNI provides high amplitude and reliable signals for improved motor control and the DS-RPNI has the potential to restore sensory feedback in individuals to allow a more functional and intuitive prosthetic device experience. The C-RPNI is a potential solution when applications of the RPNI and DS-RPNI as separate constructs are limited, including proximal transhumeral amputations. RPNI’s indications continue to expand as this technique can be applied to any transected or continuous peripheral nerve to serve as a biologically stable bioamplifier of efferent motor action potentials. The MC-RPNI is a promising neural interface to control advanced exoskeleton devices for individuals with musculoskeletal injuries. The future of these novel surgical innovative approaches will allow individuals to be able to feel and manipulate objects similar to using their native limbs and would have a much more naturalistic and intuitive experience.
DECLARATIONS
Acknowledgments
We thank Widya Adidharma and Sara Huang for providing surgical images and illustrations.
Authors’ contributions
Performed writing of the manuscript: Berberoglu I, Burke KL
Assisted with manuscript preparation: Kemp SWP, Cederna PS
Availability of data and materials
Not applicable.
Financial support and sponsorship
None.
Conflicts of interest
Dr. Paul Cederna is the president of Blue Arbor Technologies, a company which makes hardware and software to control prosthetic devices. However, none of the proprietary hardware or software offerings of the company are discussed in this paper. In addition, products of Blue Arbor Technologies are not yet available for sale on the market. All other authors declared that there are no conflicts of interest.
Ethical approval and consent to participate
The Institutional Review Board at the University of Michigan approved this study (HUM00124839). Not applicable.
Consent for publication
Not applicable.
Copyright
© The Author(s) 2024.
REFERENCES
1. Ziegler-Graham K, MacKenzie EJ, Ephraim PL, Travison TG, Brookmeyer R. Estimating the prevalence of limb loss in the United States: 2005 to 2050. Arch Phys Med Rehabil 2008;89:422-9.
2. Ma VY, Chan L, Carruthers KJ. Incidence, prevalence, costs, and impact on disability of common conditions requiring rehabilitation in the United States: stroke, spinal cord injury, traumatic brain injury, multiple sclerosis, osteoarthritis, rheumatoid arthritis, limb loss, and back pain. Arch Phys Med Rehabil 2014;95:986-95.e1.
3. Richards JT, Baird MD, Tintle SM, Souza JM, Renninger CH, Potter BK. Peripheral nerve management in extremity amputations. Orthop Clin North Am 2022;53:155-66.
5. Cowley J, Resnik L, Wilken J, Smurr Walters L, Gates D. Movement quality of conventional prostheses and the DEKA Arm during everyday tasks. Prosthet Orthot Int 2017;41:33-40.
6. Salminger S, Stino H, Pichler LH, et al. Current rates of prosthetic usage in upper-limb amputees - have innovations had an impact on device acceptance? Disabil Rehabil 2022;44:3708-13.
7. Biddiss EA, Chau TT. Upper limb prosthesis use and abandonment: a survey of the last 25 years. Prosthet Orthot Int 2007;31:236-57.
8. Burke KL, Kung TA, Hooper RC, Kemp SWP, Cederna PS. Regenerative peripheral nerve interfaces (RPNIs): current status and future direction. Plast Aesthet Res 2022;9:48.
9. Sando IC, Adidharma W, Nedic A, et al. Dermal sensory regenerative peripheral nerve interface for reestablishing sensory nerve feedback in peripheral afferents in the rat. Plast Reconstr Surg 2023;151:804e-13e.
10. Hsu E, Cohen SP. Postamputation pain: epidemiology, mechanisms, and treatment. J Pain Res 2013;6:121-36.
11. Mapplebeck JC, Beggs S, Salter MW. Molecules in pain and sex: a developing story. Mol Brain 2017;10:9.
14. Faust AE, Soletti L, Cwalina NA, et al. Development of an acellular nerve cap xenograft for neuroma prevention. J Biomed Mater Res A 2022;110:1738-48.
15. Hu Y, Ursu DC, Sohasky RA, et al. Regenerative peripheral nerve interface free muscle graft mass and function. Muscle Nerve 2021;63:421-9.
16. Ganesh Kumar N, Kung TA. Regenerative peripheral nerve interfaces for the treatment and prevention of neuromas and neuroma pain. Hand Clin 2021;37:361-71.
17. Kung TA, Langhals NB, Martin DC, Johnson PJ, Cederna PS, Urbanchek MG. Regenerative peripheral nerve interface viability and signal transduction with an implanted electrode. Plast Reconstr Surg 2014;133:1380-94.
18. Kubiak CA, Adidharma W, Kung TA, Kemp SWP, Cederna PS, Vemuri C. "Decreasing postamputation pain with the regenerative peripheral nerve interface (RPNI)". Ann Vasc Surg 2022;79:421-6.
19. Kubiak CA, Kemp SWP, Cederna PS, Kung TA. Prophylactic regenerative peripheral nerve interfaces to prevent postamputation pain. Plast Reconstr Surg 2019;144:421e-30e.
20. Kubiak CA, Kemp SWP, Cederna PS. Regenerative peripheral nerve interface for management of postamputation neuroma. JAMA Surg 2018;153:681-2.
21. Ganesh Kumar N, Kung TA, Cederna PS. Regenerative peripheral nerve interfaces for advanced control of upper extremity prosthetic devices. Hand Clin 2021;37:425-33.
22. Vu PP, Vaskov AK, Irwin ZT, et al. A regenerative peripheral nerve interface allows real-time control of an artificial hand in upper limb amputees. Sci Transl Med 2020;12:eaay2857.
23. Vu PP, Irwin ZT, Bullard AJ, et al. Closed-loop continuous hand control via chronic recording of regenerative peripheral nerve interfaces. IEEE Trans Neural Syst Rehabil Eng 2018;26:515-26.
24. Vu PP, Vaskov AK, Lee C, et al. Long-term upper-extremity prosthetic control using regenerative peripheral nerve interfaces and implanted EMG electrodes. J Neural Eng 2023;20:026039.
25. Woo SL, Kung TA, Brown DL, Leonard JA, Kelly BM, Cederna PS. Regenerative peripheral nerve interfaces for the treatment of postamputation neuroma pain: a pilot study. Plast Reconstr Surg Glob Open 2016;4:e1038.
26. Sensinger JW, Dosen S. A review of sensory feedback in upper-limb prostheses from the perspective of human motor control. Front Neurosci 2020;14:345.
27. Raspopovic S, Valle G, Petrini FM. Sensory feedback for limb prostheses in amputees. Nat Mater 2021;20:925-39.
28. Hart SE, Brown DL. Dermatosensory peripheral nerve interfaces: prevention of pain recurrence following sensory neurectomy. Hand Clin 2021;37:383-9.
29. Raspopovic S, Capogrosso M, Petrini FM, et al. Restoring natural sensory feedback in real-time bidirectional hand prostheses. Sci Transl Med 2014;6:222ra19.
30. Svientek SR, Ursu DC, Cederna PS, Kemp SWP. Fabrication of the composite regenerative peripheral nerve interface (C-RPNI) in the adult rat. J Vis Exp 2020;156:10.3791/60841.
31. Bolívar S, Udina E. Preferential regeneration and collateral dynamics of motor and sensory neurons after nerve injury in mice. Exp Neurol 2022;358:114227.
32. Svientek SR, Wisely JP, Dehdashtian A, Bratley JV, Cederna PS, Kemp SWP. The muscle cuff regenerative peripheral nerve interface for the amplification of intact peripheral nerve signals. J Vis Exp 2022;Online ahead of print.
33. Kubiak CA, Svientek SR, Dehdashtian A, et al. Physiologic signaling and viability of the muscle cuff regenerative peripheral nerve interface (MC-RPNI) for intact peripheral nerves. J Neural Eng 2021;18:0460d5.
34. Siviy C, Baker LM, Quinlivan BT, et al. Opportunities and challenges in the development of exoskeletons for locomotor assistance. Nat Biomed Eng 2023;7:456-72.
35. Tariq M, Trivailo PM, Simic M. EEG-based BCI control schemes for lower-limb assistive-robots. Front Hum Neurosci 2018;12:312.
36. Reeves J, Starbuck C, Nester C. EMG gait data from indwelling electrodes is attenuated over time and changes independent of any experimental effect. J Electromyogr Kinesiol 2020;54:102461.
37. Huang J, Huo W, Xu W, Mohammed S, Amirat Y. Control of upper-limb power-assist exoskeleton using a human-robot interface based on motion intention recognition. IEEE Trans Automat Sci Eng 2015;12:1257-70.
38. Martinez-Valdes E, Negro F, Falla D, De Nunzio AM, Farina D. Surface electromyographic amplitude does not identify differences in neural drive to synergistic muscles. J Appl Physiol 2018;124:1071-9.
39. Bowen JB, Ruter D, Wee C, West J, Valerio IL. Targeted muscle reinnervation technique in below-knee amputation. Plast Reconstr Surg 2019;143:309-12.
40. Frost CM, Ursu DC, Flattery SM, et al. Regenerative peripheral nerve interfaces for real-time, proportional control of a neuroprosthetic hand. J Neuroeng Rehabil 2018;15:108.
41. Shu T, Herrera-arcos G, Taylor CR, Herr HM. Mechanoneural interfaces for bionic integration. Nat Rev Bioeng 2024:Online ahead of print.
42. Cheesborough JE, Smith LH, Kuiken TA, Dumanian GA. Targeted muscle reinnervation and advanced prosthetic arms. Semin Plast Surg 2015;29:62-72.
43. Toyoda Y, Azoury S, Bauder A, Levin LS, Kovach S. Lower extremity amputation: the emerging role of targeted muscle reinnervation (TMR) and regenerative peripheral nerve interface (RPNI). Plast Aesthet Res 2022;9:17.
44. Mioton LM, Dumanian GA, Shah N, et al. Targeted muscle reinnervation improves residual limb pain, phantom limb pain, and limb function: a prospective study of 33 major limb amputees. Clin Orthop Relat Res 2020;478:2161-7.
45. Dumanian GA, Potter BK, Mioton LM, et al. Targeted muscle reinnervation treats neuroma and phantom pain in major limb amputees: a randomized clinical trial. Ann Surg 2019;270:238-46.
46. Van Kouwenberg EA, Chiu DTW. Adipose tissue-preserved skin grafts for lower extremity defects: recommendations to optimize outcomes. Plast Reconstr Surg Glob Open 2020;8:e2584.
47. Rinker B, Fink BF, Barry NG, et al. The effect of cigarette smoking on functional recovery following peripheral nerve ischemia/reperfusion injury. Microsurgery 2011;31:59-65.
48. Rodriguez-Fontan F, Reeves B, Tuaño K, Colakoglu S, D' Agostino L, Banegas R. Tobacco use and neurogenesis: a theoretical review of pathophysiological mechanism affecting the outcome of peripheral nerve regeneration. J Orthop 2020;22:59-63.
Cite This Article

How to Cite
Download Citation
Export Citation File:
Type of Import
Tips on Downloading Citation
Citation Manager File Format
Type of Import
Direct Import: When the Direct Import option is selected (the default state), a dialogue box will give you the option to Save or Open the downloaded citation data. Choosing Open will either launch your citation manager or give you a choice of applications with which to use the metadata. The Save option saves the file locally for later use.
Indirect Import: When the Indirect Import option is selected, the metadata is displayed and may be copied and pasted as needed.
About This Article
Special Issue
Copyright
Data & Comments
Data
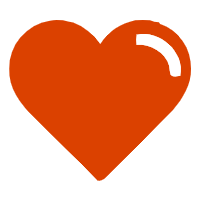
Comments
Comments must be written in English. Spam, offensive content, impersonation, and private information will not be permitted. If any comment is reported and identified as inappropriate content by OAE staff, the comment will be removed without notice. If you have any queries or need any help, please contact us at [email protected].