Repurposing multiples sclerosis disease-modifying drugs for Parkinson's disease
Abstract
Parkinson’s disease (PD) is an age-related neurodegenerative disease mainly affecting the elderly population. Despite recent progresses in pharmacologic therapies and surgical interventions such as deep brain stimulation, current PD therapies are limited to relieving disease symptoms rather than stopping disease progression, highlighting an urgent yet unmet need for disease-modifying interventions. Neuroinflammation has been proposed as a pivotal contributing factor that drives the initiation and progression of PD pathology. Owing to the revolution in disease-modifying drugs (DMDs) that successfully change the course of multiple sclerosis (MS), a central nervous system inflammatory autoimmune disease, it has become tempting to repurpose MS DMDs as new treatment options for PD. This review summarizes the ongoing and completed studies of MS DMDs in PD as a potential opportunity to address this unmet need. Future clinical trials are warranted to further evaluate the efficacy of DMDs in patients with PD.
Keywords
INTRODUCTION
Parkinson’s disease (PD) is a progressive neurodegenerative disease with motor and non-motor features. Motor features of PD are characterized by resting tremor, bradykinesia, postural instability, and rigidity[1]. Non-motor symptoms can manifest in the early stage of PD, which include hyposmia, cognitive impairment, depression, sleep disorders, autonomic dysfunction, pain, fatigue, etc.[2,3]. The selective loss of dopaminergic neurons in the substantia nigra and the presence of α-synuclein (α-syn) aggregations are considered to be the hallmarks of PD neuropathology[4]. However, the exact cause of PD remains controversial. It has been proposed that a complex interaction of aging, environment and genetics participate in the development of PD[5]. Emerging studies have prompted the link between PD and immune dysregulation. Numerous investigations have revealed that neuroinflammation is a prominent factor underlying the perpetuating neurodegeneration process of PD[6]. For example, neuroinflammation caused by SARS-CoV-2 may impair brain DA homeostasis and interfere with normal α-synuclein metabolism[7]. Aging is a strong risk factor for PD[8]. A possible explanation is that the immune disturbance occurring during the aging process, known as inflammaging, contributes to PD[9]. The dysregulation in the components of the innate immune system (e.g., microglia) and the adaptive immune system (e.g., T and B cells) in PD has also been reported by recent preclinical and clinical studies[10-12], suggesting that the pathological events in PD likely involve features seen in central nervous system (CNS) autoimmune diseases, where neuroinflammation drives disease development and progression[5].
The enhanced understanding of the link between PD and the immune system generates promising insights into the treatment of PD. Currently, the major therapy goal of PD is to provide symptomatic relief of the disease. Dopaminergic replacement pharmacotherapy (e.g., Levodopa) is the first-line therapy for PD patients[13]. However, these therapies cannot reverse the disease progression and they must be continuously maintained. After several years of treatment, fluctuations in response are inevitable[1]. Another side-effect of long-term dopaminergic treatment is levodopa-induced dyskinesias, which severely impairs the life quality of PD patients[14]. Considering the increasing health burden imposed by PD, it is imperative to develop disease-modifying therapies for PD patients. Ideally, the drugs with disease-modifying effects are able to address the factors contributing to disease progression to actually halt or even reverse the neurodegeneration process in PD. At present, no drugs used for PD have clinically proven disease-modifying effects[15]. However, in the field of CNS autoimmune diseases, particularly in multiple sclerosis (MS), disease-modifying drugs (DMDs) with immunomodulatory effects have achieved significant success. Apparently, immune dysregulation and pathological inflammatory responses are common events underlying the pathogenesis of PD and neurological autoimmune diseases. Therefore, it is reasonable to anticipate that the immunomodulatory mechanisms of action of DMDs, which are effective in neurological autoimmune diseases such as MS, may also be effective in PD. These drugs have been proven to be able to alleviate neuroinflammation to exert their neuroprotection effects. Along this line, a potentially feasible strategy is to repurpose those drugs that have been widely accepted in treating neurological autoimmune diseases to modulate the aberrant immune responses occurring in PD and ultimately achieve disease modification.
In this review, we aim to summarize the preclinical and clinical studies repurposing disease-modifying drugs for MS, or those targeting the immune system, in the treatment of PD and sum up their mechanisms. We also assessed the clinical potential of these drugs for future application in PD patients.
REPURPOSING MS DMDs FOR PD
MS is a neurological demyelinating disease caused by autoimmune responses. Demyelination and damage to axons and neurons caused by autoimmune inflammatory responses are key features of MS[16]. The application of disease-modifying treatment in MS has permanently changed the natural history and management approaches for MS. Disease-modifying drugs target specific pathways underpinning the pathology of MS to halt or even reverse the progression of the disease, rather than temporarily relieve the symptoms of the disease[15]. The prognosis of relapsing-remitting multiple sclerosis has become more favorable due to the early application of disease-modifying drugs, and there is also a trend to improve the outcome of progressive multiple sclerosis with disease-modifying therapies[17]. At present, four kinds of disease-modifying drugs for MS have been investigated in PD models [Table 1]. Notably, these drugs also function through new, unexpected pathways beyond the immune system when used in PD.
Multiple sclerosis disease-modifying drugs investigated in PD models
Drugs | Models | Mechanisms | Effects | References |
MPTP mice | Nrf2 pathway↑ NF-κB pathway↓ Anti-inflammatory function Anti-oxidant functions | Reducing behavior impartment and DA tract degeneration | [37] | |
Dimethyl fumarate | 6-OHDA mice | Nrf2 pathway↑ Anti-oxidant functions | Improvement of motor functions Reducing DA neuronal loss in the substantia nigra and striatum | [36] |
Mice receiving rAAV6-α-SYN | Nrf2 pathway↑ microgliosis and astrogliosis↓ Anti-inflammatory functions | Better motor performance Reducing DA neuron death | [34] | |
DJ-1β mutant flies | Glycolysis↑ Anti-oxidant functions | Improvement of motor functions of 5-day-old DJ-1β mutant flies | [38] | |
Transgenic A53TSyn mice | Nrf2 pathway↑ Improve mitochondrial functions Anti-oxidant functions | Improvement of neuronal arborization | [35] | |
Mice receiving rAAV6-α-SYN | CD4+T cell responses↓ | Amelioration of α-syn-induced neurodegeneration | [10] | |
MPTP mice | S1P receptor(s)-dependent Akt kinase pathway↑ | Improvement of the locomotor functions Reducing the loss of DA neurons | [41] | |
Fingolimod | 6-OHDA mice | AKT and ERK1/2 pro-survival pathway↑ BDNF↑ | Amelioration of motor deficits Protecting DA striatal neurons | [42] |
GM2+/- mice | BDNF↑ | Improvement of motor and bladder functions Reduced α-synuclein aggregation | [43] | |
transgenic A53TSyn mice | BDNF↑ | Improvement of gut motility | [44] | |
MPTP mice | NLRP3 inflammasome activation↓ ROS production↓ | Enhanced motor functions Rescuing striatal DA neuron loss | [45] | |
IFN-β | Ifnb–/– mice | Autophagy↑ | Improvement of α-synuclein clearance | [19] |
Glatiramer acetate | MPTP mice | BDNF↑ Microglial inflammation↓ | Improvement of motor functions Protecting striatal DA neurons | [28] |
IFN-β was the first approved DMD for relapsing multiple sclerosis (MS) in 1993 and is still a therapeutic option today. INF-β benefits MS patients by modulating T and B cell functions and regulating cytokine production[18]. Interestingly, IFN-β signaling in neurons has been shown to be protective against PD pathology. Ifnb–/– mice exhibit numerous characteristics of PD[19]. The deficiency in the IFN-β-IFNAR signaling axis contributed to the impairment of dopaminergic neurons, formation of Lewy Body-like structures and the presence of parkinsonism in Ifnb-/- mice, which could be rescued partly by the treatment of recombinant IFN-β[19]. However, instead of immunomodulation, further studies demonstrated that IFN-β could improve autophagy functions in neurons to facilitate the clearance of α-syn through the
Glatiramer acetate, approved for treating relapsing-remitting MS (RRMS) in 1997, induces GA-specific T cells in the periphery[22]. These T cells can be recruited to the central nervous system and express robust levels of regulatory cytokines IL-10 and TGF-β[23]. Glatiramer acetate(GA, Copaxone) was hypothesized to be beneficial to PD due to its anti-inflammatory properties[24]. Additionally, GA also facilitates the production of brain-derived neurotrophic factor (BDNF) in the brain[22,25]. Several studies demonstrated that increasing BDNF levels in the brain was effective in inhibiting PD development and BNDF was regarded as a promising target for PD therapy[26,27]. Studies regarding the impact of GA treatment on PD remain scarce. A recent study demonstrated that the treatment of GA alleviated microglial inflammation and upregulated BDNF levels in the MPTP mice brain, leading to improved movement performance[28]. However, the exact mechanisms are still waiting to be elucidated.
Dimethyl fumarate (DMF), a nuclear factor-like (Nrf2) pathway activator, received its approval for the treatment of relapsing MS in 2013[29]. This drug modulates the immune responses in MS mainly by altering the immune cell composition and preventing immune cell infiltration into CNS[29]. The mechanisms of action of DMF in MS have been reviewed in detail[30,31]. However, the role of Nrf2 pathway in PD remains controversial. The enhanced systemic activation of Nrf2 was observed in blood leukocytes of PD patients recently, revealing a potential pathological role of the Nrf2-pathway in PD[32]. However, the neuroprotective functions of Nrf2 pathway in PD have also been reported[33]. In addition, a growing body of evidence suggests that DMF is a promising candidate for PD therapy, supporting the protective role of Nrf2 axis[34,35].
Multiple mechanisms of action for this drug have been identified in different types of PD animal models. An in vitro and in vivo study demonstrated that treatment of DMF resulted in an upregulated anti-oxidant gene expression in SH-SY5Y cells and reduced oxidative stress damage in 6-OHDA mice[36]. In mice injected with rAAV6-α-SYN, DMF treatment dampened microgliosis and astrogliosis[34]. The anti-inflammatory and anti-oxidant functions of DMF were further proved by a study on the MPTP mice model, which highlighted that DMF not only exerted its anti-oxidant functions via Nrf2-mediated pathway but also displayed
Fingolimod (FTY720) was approved for treating relapsing-remitting multiple sclerosis due to its effective immunosuppressive functions[39,40]. Fingolimod is phosphorylated by Sphingosine kinase in vivo to generate fingolimod-P, which competes with S1P and induces internalization and degradation of S1P1 receptors, resulting in functional antagonism of S1P1[39]. The downregulation of S1P1 on T cells impairs its ability to egress out of secondary lymphoid organs, preventing pathogenic T cells from entering the CNS[39,40]. Similarly, suppression of T cell responses by fingolimod also confers protection against PD pathology[10]. In addition to immunomodulation functions, the neuroprotective functions of fingolimod can mainly be attributed to the stimulation of S1P receptor pathways and/or inducing the production of BDNF in the CNS[41-44]. Another study employing the MPTP mice model revealed that NLRP3 inflammasome activation, which is associated with PD development, could be inhibited by fingolimod[45]. However, there are existing controversies regarding the protective effects of fingolimod in PD models[46]. Notably, except for locomotor abilities, treatment of fingolimod also improves other PD-related symptoms, including urinary functions and gut motility[43,44]. Considering urinary dysfunctions and constipation precede the development of motor symptoms of PD, the use of fingolimod has the potential to halt PD progression in the early stage.
REPURPOSING OTHER DRUGS WITH IMMUNOMODULATORY EFFECTS FOR PD
Although the studies of disease-modifying drugs for MS are still in the preclinical stage, several clinical studies have aimed to validate the feasibility and evaluate the benefits of repositioning other types of drugs targeting the immune system for PD patients [Table 2].
Repurposing other drugs with immune modulatory effects for PD
Drugs | Original use | Mechanisms of action | NCT number | Phase | Status | Start date | Publications |
NCT01882010 | 1 | Completed | 2013 | [48] | |||
Sargramostim | Growth factor for leukocytes therapy | Treg cells | NCT03790670 | 1 | Active, not recruiting | 2019 | [49] |
NCT05677633 | 1 | Not yet recruiting | 2023 | - | |||
Simvastatin | Cholesterol control | Anti-inflammation | NCT02787590/ISRCTN16108482 | 2 | Completed | 2016 | [54,65] |
Azathioprine | Immunosuppression | Suppression of the peripheral immunity | ISRCTN14616801/EudraCT- 2018-003089-14 | 2 | Active, not recruiting | 2018 | [59] |
Sargramostim, a recombinant human granulocyte-macrophage colony-stimulating factor (GM-CSF), has been approved for use in the recovery of myeloid function and anti-cancer therapies, such as melanoma, due to its abilities to promote bone marrow regeneration and modulate the immune system[47]. In a randomized, double-blind phase 1 clinical trial, patients receiving 6 μg/kg/day sargramostim for six weeks showed modest improvement in motor functions measured by MDS-UPDRS-III score, which was associated with the concomitant improvement of Treg numbers and function[48]. A further study proposed 3 μg/kg/day as a more optimal dose with higher tolerability and fewer adverse events[49]. The absence of a reliable biomarker to monitor disease progression and therapeutic efficacy has been a persistent issue waiting to address in the treatment of PD. This issue has impeded the development of effective treatments and made it difficult for clinicians to accurately evaluate the effectiveness of current therapies. A recent study analyzed transcriptomic and proteomic data from monocytes of Parkinson’s disease patients undergoing sargramostim treatment. The findings indicated that the monocyte profile could serve as a potential biomarker for assessing the therapeutic response to sargramostim of PD patients[50]. These results provide important insights into the mechanisms of action of sargramostim and could lead to improved treatment strategies for Parkinson's disease.
Simvastatin belongs to the class of statin drugs, which reduce blood cholesterol levels by inhibiting
Azathioprine, which interferes with nucleic acid metabolism to inhibit immune cell proliferation, is a widely used immunosuppressant drug in autoimmune diseases[55]. Specifically, azathioprine is still used as the
In summary, ongoing clinical trials are testing the potential of the aforementioned drugs with immune modulatory effects in patients with PD. The new results in the coming years may provide evidence to extend the original pharmaceutical scope of these old drugs for clinical use in PD.
CONCLUSION AND FUTURE PERSPECTIVES
Owing to the aging demographic structure of the global population, the prevalence of PD has grown exponentially in the past decades[60]. In the absence of effective disease-modifying therapy, the subsequent increasing health burden imposed by PD is becoming an issue that cannot be ignored. Treatment options for MS have been tremendously improved over the past 20 years. The success of these newly developed oral DMDs and monoclonal antibodies in MS and their various mechanisms of action have led to active investigations to test their efficacy in PD and other age-related neurological disorders[15,61].
Mounting evidence has indicated neuroinflammation as a pivotal contributor that drives the initiation and progression of PD pathology[62]. Ongoing clinical trials are being carried out to explore whether repurposing drugs with immunomodulatory effects could be a viable approach to benefit patients with PD, although current clinical studies are still limited to the early phase. Considering the success of DMDs in MS to control CNS inflammation and their favorable features of pharmacokinetics and tissue distribution, it is reasonable to postulate their efficacy in modulating the inflammatory responses in the brain, which might benefit patients with PD. Furthermore, repurposing approved drugs is a more time and cost-efficient approach compared to the de novo development of a new agent[63].
Multiple mechanisms of MS DMDs have been identified in PD animal models [Figure 1]. Among MS DMDs, DMF and fingolimod have been demonstrated to generate positive outcomes in a wide array of PD models via various mechanisms, suggesting that they are promising candidates to be tested in future clinical trials. Additionally, we should keep in mind that the newly developed DMDs such as Bruton tyrosine kinase inhibitors might also be promising candidates for future experimental studies in PD[64]. Future investigations regarding these drugs may lead to the identification of promising new therapies for PD and other neurodegenerative disorders that represent a significant unmet clinical need.
DECLARATIONS
Author’s contribution
Provided the idea: Zhu X, Liu Q
Wrote the manuscript: Cao T
Availability of data and materials
Not applicable.
Financial support and sponsorship
This work was funded by Tianjin Key Medical Discipline (Specialty) Construction Project, Tianjin Health Commission under grants (TJWJ2022MS004 and 2023001), Tianjin Municipal Science and Technology Commission (22YDTPJC00350).
Conflicts of interest
The author declared that there are no conflicts of interest.
Ethical approval and consent to participate
Not applicable.
Consent for publication
Not applicable.
Copyright
© The Author(s) 2023.
REFERENCES
3. Schapira AHV, Chaudhuri KR, Jenner P. Non-motor features of Parkinson disease. Nat Rev Neurosci 2017;18:509.
4. Dehay B, Bourdenx M, Gorry P, et al. Targeting α-synuclein for treatment of Parkinson’s disease: mechanistic and therapeutic considerations. Lancet Neurol 2015;14:855-66.
5. Tansey MG, Wallings RL, Houser MC, Herrick MK, Keating CE, Joers V. Inflammation and immune dysfunction in Parkinson disease. Nat Rev Immunol 2022;22:657-73.
6. Lee SYH, Yates NJ, Tye SJ. Inflammatory mechanisms in Parkinson’s disease: from pathogenesis to targeted therapies. Neuroscientist 2022;28:485-506.
7. Mancini M, Natoli S, Gardoni F, Di Luca M, Pisani A. Dopamine transmission imbalance in neuroinflammation: perspectives on long-term COVID-19. Int J Mol Sci 2023;24:5618.
8. Vila M. Neuromelanin, aging, and neuronal vulnerability in Parkinson’s disease. Mov Disord 2019;34:1440-51.
9. Calabrese V, Santoro A, Monti D, et al. Aging and Parkinson’s disease: inflammaging, neuroinflammation and biological remodeling as key factors in pathogenesis. Free Radic Biol Med 2018;115:80-91.
10. Williams GP, Schonhoff AM, Jurkuvenaite A, Gallups NJ, Standaert DG, Harms AS. CD4 T cells mediate brain inflammation and neurodegeneration in a mouse model of Parkinson’s disease. Brain 2021;144:2047-59.
11. Bartels T, De Schepper S, Hong S. Microglia modulate neurodegeneration in Alzheimer’s and Parkinson’s diseases. Science 2020;370:66-9.
12. Li R, Tropea TF, Baratta LR, et al. Abnormal B-Cell and Tfh-Cell profiles in patients with Parkinson disease: a cross-sectional study. Neurol Neuroimmunol Neuroinflamm 2021;9:e1125.
13. Armstrong MJ, Okun MS. Diagnosis and treatment of Parkinson disease: a review. JAMA 2020;323:548-60.
14. Chagraoui A, Boulain M, Juvin L, Anouar Y, Barrière G, Deurwaerdère P. L-DOPA in Parkinson’s disease: looking at the “false” neurotransmitters and their meaning. Int J Mol Sci 2019;21:294.
15. Vijiaratnam N, Simuni T, Bandmann O, Morris HR, Foltynie T. Progress towards therapies for disease modification in Parkinson’s disease. Lancet Neurol 2021;20:559-72.
16. Wiendl H, Gold R, Berger T, et al. “Multiple Sclerosis Therapy Consensus Group” (MSTCG). Multiple sclerosis therapy consensus group (MSTCG): position statement on disease-modifying therapies for multiple sclerosis (white paper). Ther Adv Neurol Disord 2021;14:17562864211039648.
17. Sorensen PS, Sellebjerg F, Hartung HP, Montalban X, Comi G, Tintoré M. The apparently milder course of multiple sclerosis: changes in the diagnostic criteria, therapy and natural history. Brain 2020;143:2637-52.
18. Jakimovski D, Kolb C, Ramanathan M, Zivadinov R, Weinstock-Guttman B. Interferon β for multiple sclerosis. Cold Spring Harb Perspect Med 2018;8:a032003.
19. Ejlerskov P, Hultberg JG, Wang J, et al. Lack of neuronal IFN-β-IFNAR causes Lewy body- and Parkinson’s disease-like dementia. Cell 2015;163:324-39.
20. Ejlerskov P, Rubinsztein DC, Pocock R. IFNB/interferon-β regulates autophagy via a MIR1-TBC1D15-RAB7 pathway. Autophagy 2020;16:767-9.
21. Roessler HI, Knoers NVAM, van Haelst MM, van Haaften G. Drug repurposing for rare diseases. Trends Pharmacol Sci 2021;42:255-67.
22. Ziemssen T, Kümpfel T, Klinkert WEF, Neuhaus O, Hohlfeld R. Glatiramer acetate-specific T-helper 1- and 2-type cell lines produce BDNF: implications for multiple sclerosis therapy. Brain-derived neurotrophic factor. Brain 2002;125:2381-91.
23. Aharoni R. The mechanism of action of glatiramer acetate in multiple sclerosis and beyond. Autoimmun Rev 2013;12:543-53.
24. Tsai SJ. Glatiramer acetate could be a potential therapeutic agent for Parkinson‘s disease through its neuroprotective and anti-inflammatory effects. Medical Hypotheses 2007;69:1219-21.
25. Mahurkar S, Suppiah V, O’Doherty C. Pharmacogenomics of interferon beta and glatiramer acetate response: a review of the literature. Autoimmun Rev 2014;13:178-86.
26. Ahn EH, Kang SS, Liu X, et al. BDNF and Netrin-1 repression by C/EBPβ in the gut triggers Parkinson’s disease pathologies, associated with constipation and motor dysfunctions. Prog Neurobiol 2021;198:101905.
27. Palasz E, Wysocka A, Gasiorowska A, Chalimoniuk M, Niewiadomski W, Niewiadomska G. BDNF as a promising therapeutic agent in Parkinson’s disease. Int J Mol Sci 2020;21:1170.
28. Churchill MJ, Cantu MA, Kasanga EA, Moore C, Salvatore MF, Meshul CK. Glatiramer acetate reverses motor dysfunction and the decrease in tyrosine hydroxylase levels in a mouse model of Parkinson’s disease. Neuroscience 2019;414:8-27.
29. Montes Diaz G, Hupperts R, Fraussen J, Somers V. Dimethyl fumarate treatment in multiple sclerosis: recent advances in clinical and immunological studies. Autoimmun Rev 2018;17:1240-50.
31. Yang JH, Rempe T, Whitmire N, Dunn-Pirio A, Graves JS. Therapeutic advances in multiple sclerosis. Front Neurol 2022;13:824926.
32. Petrillo S, Schirinzi T, Di Lazzaro G, et al. Systemic activation of Nrf2 pathway in Parkinson’s disease. Mov Disord 2020;35:180-4.
33. Zhang C, Zhao M, Wang B, et al. The Nrf2-NLRP3-caspase-1 axis mediates the neuroprotective effects of celastrol in Parkinson’s disease. Redox Biol 2021;47:102134.
34. Lastres-Becker I, García-Yagüe AJ, Scannevin RH, et al. Repurposing the NRF2 activator dimethyl fumarate as therapy against synucleinopathy in Parkinson’s disease. Antioxid Redox Signal 2016;25:61-77.
35. Brandes MS, Zweig JA, Tang A, Gray NE. NRF2 activation ameliorates oxidative stress and improves mitochondrial function and synaptic plasticity, and in A53T α-synuclein hippocampal neurons. Antioxidants 2021;11:26.
36. Jing X, Shi H, Zhang C, et al. Dimethyl fumarate attenuates 6-OHDA-induced neurotoxicity in SH-SY5Y cells and in animal model of Parkinson’s disease by enhancing Nrf2 activity. Neuroscience 2015;286:131-40.
37. Campolo M, Casili G, Biundo F, et al. The Neuroprotective effect of dimethyl fumarate in an MPTP-mouse model of Parkinson’s disease: involvement of reactive oxygen species/nuclear factor-κB/nuclear transcription factor related to NF-E2. Antioxid Redox Signal 2017;27:453-71.
38. Solana-Manrique C, Sanz FJ, Ripollés E, et al. Enhanced activity of glycolytic enzymes in drosophila and human cell models of Parkinson’s disease based on DJ-1 deficiency. Free Radic Biol Med 2020;158:137-48.
39. Huwiler A, Zangemeister-Wittke U. The sphingosine 1-phosphate receptor modulator fingolimod as a therapeutic agent: recent findings and new perspectives. Pharmacol Ther 2018;185:34-49.
40. Chun J, Kihara Y, Jonnalagadda D, Blaho VA. Fingolimod: lessons learned and new opportunities for treating multiple sclerosis and other disorders. Annu Rev Pharmacol Toxicol 2019;59:149-70.
41. Motyl J, Przykaza Ł, Boguszewski PM, Kosson P, Strosznajder JB. Pramipexole and fingolimod exert neuroprotection in a mouse model of Parkinson’s disease by activation of sphingosine kinase 1 and akt kinase. Neuropharmacology 2018;135:139-50.
42. Ren M, Han M, Wei X, et al. FTY720 attenuates 6-OHDA-associated dopaminergic degeneration in cellular and mouse Parkinsonian models. Neurochem Res 2017;42:686-96.
43. Vidal-Martinez G, Najera K, Miranda JD, et al. FTY720 improves behavior, increases brain derived neurotrophic factor levels and reduces α-synuclein pathology in Parkinsonian GM2+/- mice. Neuroscience 2019;411:1-10.
44. Vidal-Martínez G, Vargas-Medrano J, Gil-Tommee C, et al. FTY720/fingolimod reduces synucleinopathy and improves gut motility in A53T mice: contributions of pro-brain-derived neurotrophic factor (pro-bdnf) and mature bdnf. J Biol Chem 2016;291:20811-21.
45. Yao S, Li L, Sun X, et al. FTY720 Inhibits MPP+-induced microglial activation by affecting NLRP3 inflammasome activation. J Neuroimmune Pharmacol 2019;14:478-92.
46. Komnig D, Dagli TC, Habib P, Zeyen T, Schulz JB, Falkenburger BH. Fingolimod (FTY720) is not protective in the subacute MPTP mouse model of Parkinson’s disease and does not lead to a sustainable increase of brain-derived neurotrophic factor. J Neurochem 2018;147:678-91.
47. Lazarus HM, Ragsdale CE, Gale RP, Lyman GH. Sargramostim (rhu GM-CSF) as cancer therapy (systematic review) and an immunomodulator. A drug before its time? Front Immunol 2021;12:706186.
48. Gendelman HE, Zhang Y, Santamaria P, et al. Evaluation of the safety and immunomodulatory effects of sargramostim in a randomized, double-blind phase 1 clinical Parkinson’s disease trial. NPJ Parkinsons Dis 2017;3:10.
49. Olson KE, Namminga KL, Lu Y, et al. Safety, tolerability, and immune-biomarker profiling for year-long sargramostim treatment of Parkinson’s disease. EBioMedicine 2021;67:103380.
50. Abdelmoaty MM, Machhi J, Yeapuri P, et al. Monocyte biomarkers define sargramostim treatment outcomes for Parkinson’s disease. Clin Transl Med 2022;12:e958.
51. Eshaghi A, Kievit RA, Prados F, et al. Applying causal models to explore the mechanism of action of simvastatin in progressive multiple sclerosis. Proc Natl Acad Sci U S A 2019;116:11020-7.
52. Carroll CB, Wyse RKH. Simvastatin as a potential disease-modifying therapy for patients with Parkinson’s disease: rationale for clinical trial, and current progress. J Parkinsons Dis 2017;7:545-68.
53. Wolozin B, Wang SW, Li NC, Lee A, Lee TA, Kazis LE. Simvastatin is associated with a reduced incidence of dementia and Parkinson’s disease. BMC Med 2007;5:20.
54. Stevens KN, Creanor S, Jeffery A, et al. PD STAT Study Group. Evaluation of simvastatin as a disease-modifying treatment for patients with Parkinson disease: a randomized clinical trial. JAMA Neurol 2022;79:1232-41.
55. Broen JCA, van Laar JM. Mycophenolate mofetil, azathioprine and tacrolimus: mechanisms in rheumatology. Nat Rev Rheumatol 2020;16:167-78.
56. Zhang C, Zhang M, Qiu W, et al. TANGO Study Investigators. Safety and efficacy of tocilizumab versus azathioprine in highly relapsing neuromyelitis optica spectrum disorder (TANGO): an open-label, multicentre, randomised, phase 2 trial. Lancet Neurol 2020;19:391-401.
57. Holmøy T, Høglund RA, Illes Z, Myhr KM, Torkildsen Ø. Recent progress in maintenance treatment of neuromyelitis optica spectrum disorder. J Neurol 2021;268:4522-36.
58. Chan KH, Lee CY. Treatment of neuromyelitis optica spectrum disorders. Int J Mol Sci 2021;22:8638.
59. Greenland JC, Cutting E, Kadyan S, Bond S, Chhabra A, Williams-Gray CH. Azathioprine immunosuppression and disease modification in Parkinson’s disease (AZA-PD): a randomised double-blind placebo-controlled phase II trial protocol. BMJ Open 2020;10:e040527.
60. Qi S, Yin P, Wang L, et al. Prevalence of Parkinson’s disease: a community-based study in China. Mov Disord 2021;36:2940-4.
61. McFarthing K, Rafaloff G, Baptista M, et al. Parkinson’s disease drug therapies in the clinical trial pipeline: 2022 update. J Parkinsons Dis 2022;12:1073-82.
62. MacMahon Copas AN, McComish SF, Fletcher JM, Caldwell MA. The Pathogenesis of Parkinson’s disease: a complex interplay between astrocytes, microglia, and t lymphocytes? Front Neurol 2021;12:666737.
63. Chen X, Gumina G, Virga KG. Recent advances in drug repurposing for Parkinson’s disease. Curr Med Chem 2019;26:5340-62.
64. Abdi IY, Bartl M, Dakna M, et al. Cross-sectional proteomic expression in Parkinson’s disease-related proteins in drug-naïve patients vs healthy controls with longitudinal clinical follow-up. Neurobiol Dis 2023;177:105997.
Cite This Article
Export citation file: BibTeX | RIS
OAE Style
Cao T, Liu Q, Zhu X. Repurposing multiples sclerosis disease-modifying drugs for Parkinson's disease. Ageing Neur Dis 2023;3:11. http://dx.doi.org/10.20517/and.2023.08
AMA Style
Cao T, Liu Q, Zhu X. Repurposing multiples sclerosis disease-modifying drugs for Parkinson's disease. Ageing and Neurodegenerative Diseases. 2023; 3(2): 11. http://dx.doi.org/10.20517/and.2023.08
Chicago/Turabian Style
Cao, Tingyu, Qiang Liu, Xiaodong Zhu. 2023. "Repurposing multiples sclerosis disease-modifying drugs for Parkinson's disease" Ageing and Neurodegenerative Diseases. 3, no.2: 11. http://dx.doi.org/10.20517/and.2023.08
ACS Style
Cao, T.; Liu Q.; Zhu X. Repurposing multiples sclerosis disease-modifying drugs for Parkinson's disease. Ageing. Neur. Dis. 2023, 3, 11. http://dx.doi.org/10.20517/and.2023.08
About This Article
Copyright
Data & Comments
Data
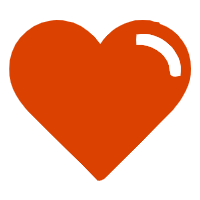

Comments
Comments must be written in English. Spam, offensive content, impersonation, and private information will not be permitted. If any comment is reported and identified as inappropriate content by OAE staff, the comment will be removed without notice. If you have any queries or need any help, please contact us at support@oaepublish.com.