Contemporary approach to heavily calcified lesions: tools of the trade, challenges, and pitfalls
Abstract
Coronary artery calcifications (CAC) affect more than 90% of men and more than 67% of women older than 70; the spread is mainly due to the high occurrence of major cardiovascular risk factors. The presence of CAC can be detected by several noninvasive and invasive methods like computed tomography (CT), coronary angiography (CA), Intravascular Ultrasound (IVUS), and Optical Coherence Tomography (OCT), with each system providing different information that can be used in the treatment strategy of CAC. Several devices can modify calcium during PCI: high-pressure non-compliant balloons, cutting/scoring balloons, atheroablative technologies, and intravascular Lithotripsy (IVL). Each technique has advantages and disadvantages that every interventional cardiologist should know to perform an optimal PCI and to achieve the best result and clinical outcome. This is a narrative review that aims to illustrate the contemporary management of CAC, focusing on the available techniques to assess calcifications and their novel advancements and explaining the existing tools to treat CAC with a focus on their significant challenges and pitfalls.
Keywords
INTRODUCTION
Coronary artery calcifications (CAC) are increasing because of the high prevalence of risk factors and advanced age. The bulk of moderate to severe CAC in the population undergoing percutaneous coronary intervention (PCI) is estimated to be around 32%, of which 6%-20% is considered severe[1]. The availability of different and ever more sophisticated systems to detect CAC, together with the development of several tools able to modify calcium, allows treating calcific stenosis and performing PCI in most patients. Recent studies demonstrate PCI optimal results and better outcomes, also in the long term. This narrative review examines CAC’s contemporary assessment and treatment, focusing on the pitfalls and challenges of debulking calcific lesions.
ASSESSMENT OF CORONARY ARTERY CALCIFICATIONS
Coronary computed tomography angiography
Coronary computed tomography angiography (CCTA) is the principal noninvasive imaging technique used to spot calcium. It has high diagnostic accuracy for identifying coronary artery disease (CAD), pivotal in managing patients with low-to-intermediate pre-test likelihood of CAD[2] and in patients with stable chest pain, as demonstrated by the SCOT-HEART study[3]. Calcium is identified as a zone of hyper attenuation, determined by at least one mm2 with > 130 Hounsfield units or ≥ three adjacent pixels using the Agatston method[4] [Figure 1]. Non-contrast CT scan offers the possibility of obtaining coronary artery calcium scoring (CACS), which has a robust prognostic value in the mid to long term in asymptomatic individuals[5]. CCTA can accurately quantify coronary stenosis severity (volume and extension) and characterize coronary atherosclerosis by detecting spotty calcification; the latter, together with low CT attenuation, remarkable positive remodeling, and the “napkin-ring sign”, represents one of the four signs of vulnerable plaques[6]. Regarding spotty calcification, several studies have demonstrated the key role of 18F-NaF Positron Emission Tomography (PET) in identifying the microcalcification[7], as a marker of active mineral deposition in the atherosclerotic plaque[8]. Therefore, this tracer can be useful in detecting vulnerable plaque[9] and patients with elevated CAD risk[8,10].
Figure 1. Coronary computed coronary angiography with (A) and without (B) contrast media injection. Calcium deposits show up as bright white spots in coronary vessels (red arrow).
However, extensive coronary calcification may compromise image quality due to blooming and beam-hardening or “streak” artifacts, resulting in under- and overestimation of lumen stenosis[2]; these historical limitations are being potentially challenged with emerging technologies[11], from subtraction technique[12] to artificial intelligence software. A recent study demonstrated that the Enhanced Super-Resolution Generative Adversarial Network (ESRGAN) could suppress the blooming artifact improving the specificity and Positive Predictive Value of CCTA[13]; another study has shown a significant impact of Virtual Monoenergetic Imaging and Quantum Iterative Reconstructions in the accuracy of CACS, using a virtual noniodine reconstruction algorithm on a first-generation, clinical, photon counting detector CT system[14].
Therefore, CCTA could help to plan and guide PCI procedures involving CAC by calculating calcium score and calcific plaque distribution, identifying lesions requiring additional techniques to modify calcium[15]; recently, Kurogi et al. indicated that an elevated mean density of the cross-sectional CT image was the strongest predictor of the need for Rotational Atherectomy (RA) during PCI[16]. In the field of Chronic Total Occlusion (CTO), CCTA can recognize anatomical characteristics that are potentially able to augment the difficulty of CTO PCI (calcium > 50% cross-sectional area at the point of CTO maximum calcification, calcification at CTO entry point, calcification length > 5.5 mm), as also revealed by the PROGRESS-CTO registry[17]; it can be useful to calculate newer CCTA derived scores based on calcium morphology and extension, to predict procedural success[15,18], which could replace the actual angiographic scores, like Japan-CTO (J-CTO) score. Although the scope of this review is not to provide a detailed classification of CTO, it is worth mentioning that Yamasaki et al. from Osaka University had recently introduced a classification for calcium lesions assessed by Multi-slice CT[19], distinguishing calcified plaque in bean, stone, rock, ring and full moon-like; Panuccio et al. had recently demonstrated that “Full Moon”- like calcification is commonly detected in CTO and it has a predictive value for complexity during CTO-PCI[20].
Coronary angiography
Coronary angiography (CA) has conventionally been used to assess and quantify CAC using fluoroscopy methods. In particular, a radiopacity detected only during the cardiac cycle before injection of the contrast dye, identifies a moderate calcification; a radiopacity detected without cardiac motion, visible on both sides of the arterial lumen, as a double track[21], identifies a severe or heavy calcification [Figure 2]. In this manuscript, the latter terms are similar. Fluoroscopy is very specific (98.7%) for detecting severe CAC, predicting worse stent expansion in these cases[22]. Still, its sensibility is low (40%-48%) compared to other intravascular imaging modalities, as demonstrated by a study conducted by Wang et al. CA often underestimates calcium, it is not able to measure calcium depth and its grading is inaccurate[23].
Figure 2. Severe calcification as “double track” radiopacity of Left Anterior Descending coronary artery, detected by coronary angiography (white arrow).
Nowadays, several technologically advanced tools such as add-on diagnostic CA are spreading; among these ones, the CoroFinder developed by Zaffino et al. deserves a special mention because of its ability to automatically identify coronary tree borders and to dynamically track the vessel position without contrast agents[24]. Also, the Dynamic Coronary Roadmap (DCM) (Philips, Amsterdam, Europe) is a commercial tool able to automatically generate a real-time, dynamic view of coronary arteries on fluoroscopy that can be used for navigation during PCI[25]. These innovative technologies could have a potential application in the field of CAC identification and quantification.
Intravascular ultrasound
Intravascular Ultrasound (IVUS) is an intravascular imaging technique that can detect calcium as brilliant, hyperechoic lines with acoustic shadowing, discriminating in superficial (close to the lumen) and deep [Figure 3]; thanks to the elevated penetration power of ultrasound, it can see deep calcium, with 150 to
Figure 3. Detection of coronary calcium by IntraVascular ultraSound (IVUS). (A) shows superficial calcium protruding into the vessel lumen (white arrow). A calcium arc > 180° often results in a tight stenosis that can hardly be dilated without appropriate debulking (B). The red arrow in the (C) indicates a deep calcification.
IVUS can semiquantitatively assess calcified plaques, measuring calcium arc and length and estimating the calcific burden. It can also identify calcified nodules (CN) and nodular calcification.
The limitations of IVUS are represented by the inability to detect the thickness of calcium and microcalcifications or if these are placed underneath large necrotic cores; however, calcium usually produces posterior echo reverberations, a very distinctive feature[21,22].
IVUS can guide PCI of CAC from the beginning, with morphologic and semiquantitative calcium assessment, to the end of the procedure, checking the result post-stent implantation. In several prospective and retrospective multi-center trials, IVUS-guided PCI has demonstrated better clinical outcomes than angiography-guided PCI[28], especially for complex PCI[29].
Pre-intervention evaluation is essential to plan the procedure because assessing the calcium subset could guide the plaque modification strategy, the choice for the debulking system, and the correct vessel sizing.
Zhang et al. recently reported an IVUS score for calcified lesions: a superficial calcium arc of > 270° longer than 5 mm, a superficial calcium arc of 360°, the identification of a CN, and a vessel diameter of < 3.5 mm correlated with stent underexpansion[30]; they suggested the use of debulking technique if calcium score is
IVUS could also assess adequate vessel preparation. In this setting, the reverberation phenomenon is frequently observed for severely calcified lesions after rotational atherectomy (RA). It seems to be due to the smooth inner surfaces of calcifications and has been proposed as a surrogate indicator of thinner calcium. Recently, You et al. reported that the number of layers of reverberation signal identified by IVUS is positively associated with the calcifications’ thickness quantified by OCT after RA, and calcium crack after predilatation produces a single layer of reverberation[31].
After stent implantation, IVUS can assess procedural success, measure the stent lumen, check adequate stent expansion, and identify edges dissection and incomplete stent apposition[32].
Optical coherence tomography
On optical coherence tomography (OCT) imaging, calcium appears thin, with a low-intensity signal area and well-delineated sharp borders[21] [Figure 4]. Thanks to its higher resolution (10 to 20 µm), OCT is able to quantify calcium thickness and show calcium disruption; its sensitivity and specificity for CAC are
Figure 4. Calcification detected by optical coherence tomography (OCT) as signal-poor area with well-delineated external contours (A). Nodular calcification detected by OCT (B). OCT reveals calcium fracture (red arrow) after the use of intravascular lithotripsy (C).
Figure 5. The OCT software (UltreonTM, Abbott, Illinois, US) is able to automatically measure the maximum calcium thickness and total angle of calcium (A). OCT can check the result, automatically calculating stent expansion and minimal stent area (B).
A recent study conducted by Fujino et al. presented an OCT score to classify calcium plaques with a numerical cut-off; calcium arc > 180° (2 points), calcium length
OCT disadvantages include injecting contrast medium to minimize light scatter from red blood cells, the possibility of missing deep calcifications due to insufficient penetration[21], and the potential misinterpretation of necrotic cores as calcium deposits[22].
Like IVUS, OCT is helpful in the planning of PCI, to choose a possible adjunctive intracoronary device dedicated to modify CAC by ablating its surface and breaking its integrity (fractures and discontinuities)[22]. OCT can check the effects of calcium debulking, identifying the presence of calcium fractures, which seem to be related to larger stent expansion and better procedural outcomes. The results of two recent studies suggested that CAC with extensive circumferential length and minor minimum axial thickness tended more to crack after conventional PCI[35,36]; these findings supported a dominant role of OCT in determining which calcium plaques need additional lesion preparation.
Finally, OCT can assess post-stent implantation by checking the presence of stent malapposition, measuring the distance between the abluminal surface of the strut and the luminal surface of the artery wall[37], edge dissections, and stent expansion [Figure 5B].
CURRENT INTERVENTIONAL APPROACH TO CALCIFIED LESIONS
PCI for calcified stenosis can be performed by trans-femoral or trans-radial approach, depending principally on operator experience[21]. Strategies that can facilitate the treatment of CAC include support wires, buddy wires, guide extensions, and lesion predilatation[38,39]. When these options are not able to achieve an adequate treatment, a successful lesion preparation could be realized using a dedicated device to simplify stent delivery, enhance final stent expansion and apposition, reduce procedural complications, and improve clinical outcomes[40].
Cutting, scoring, and high-pressure balloon
Cutting balloons (CB) consist of three or four metal microblades located longitudinally on the surface. The balloon should be inflated sequentially first to 2 atmospheres (atm), next 4 atm, and at last to nominal pressure (NP) 6 atm; it works by cutting the media through endovascular radial incisions in the fibrocalcific tissue, resulting in larger expansion than traditional balloons and minimizing neointima proliferation[21]. Also, the blades anchor into the intima, thus avoiding balloon slippage, often observed in in-stent restenosis (ISR)[39]. An IVUS-based study conducted by Okura et al. demonstrated that CB angioplasty achieved more lumen enlargement than conventional balloon angioplasty[41]. Several observational studies confirmed this finding, also showing that CB angioplasty was associated with higher final minimal stent area (MSA)[42]; the recent COPS randomized trial has demonstrated that treatment with CB inflated at high atm is related to larger MSA and superior eccentricity of the stent at the level of the calcium site, compared with non-compliant (NC) balloon[43].
Scoring balloons are encircled by nitinol spiral wires, which work through intimo-medial dissection, as demonstrated by OCT[44]. This mechanism of action allows the focal concentration of the force, resulting in the achievement of full expansion with low atm and subsequently less vessel injury, less risk of coronary dissections, and less balloon slippage[45,46]. Scoring balloons have analogous indications to CB, but they are more flexible and have a better profile. Nowadays, there are different kinds of scoring balloons for the treatment of CAC: the AngioSculpt (Spectranetics-Philips) is a semi-compliant balloon consisting of three spiral rectangular nitinol scoring components; it also exists in a drug-coated form (AngioSculpt X, Spectranetics-Philips)[47]; the Scoreflex (OrbusNeich Medical, China) is an NC balloon with a dual-wire system. A study conducted by De Ribamar Costa et al. suggested that the predilatation with a scoring balloon could improve Drug-eluting stent (DES) expansion[48], but data on their use in calcific lesions are still limited. A small case series published by Otsuka et al. showed that prolonged inflation of a Scoreflex balloon permits an effective preparation of a severely calcified lesion that was unresponsive to traditional systems with or without RA, probably by the “creep phenomenon”, through which sustained tensile stress produces a distortion of heavily calcium[49]. Complications with scoring balloons are relatively infrequent; a recent study has shown that with AngioSculpt, complications mainly happened in the severely calcified plaques located in the Left Anterior Descending (LAD) artery and are related to device tip break and complexity upon withdrawal[50].
High-Pressure balloon such as OPN-NC (SIS Medical AG, Winterthur, Switzerland) is a dual-layer balloon that permits inflation to extreme pressure (35 atm) with uniform expansion, which may modify calcium. Secco et al. first reported the success of OPN in un-expandable stents due to CAC, ISR, and de novo heavily calcified plaques where classic NC balloon failed[51]; in a retrospective study, they demonstrated OPN safety and procedural success (96.6%) in 326 un-dilatable lesions treated with inflation up to 45-50 atm, with only 3 cases of coronary perforations[52]. The ISAR-CALC randomized trial conducted by Rheude et al. compared stent expansion after predilatation of heavily calcified plaques with either an extreme high-pressure balloon or a scoring balloon: data showed comparable stent expansion on OCT, with increased minimum lumen diameter and reduced diameter stenosis by super high-pressure balloon[53]. Results from the ISAR-CALC 2 trial are expected; this study aims to demonstrate the superiority of an extreme high-pressure balloon compared to the intravascular lithotripsy, in the preparation of severely calcified un-dilatable coronary plaques before DES implantation[54]. Contrary to common opinion, the risk of perforation using OPN balloons is low. A recent multi-center retrospective OCT registry, including 50 instances of CAC treated with OPN NC alone or after other devices if needed, confirmed acceptable expansion with one flow limiting dissection needing stent implantation and three non-cardiovascular deaths in 6 months follow-up. No perforation, no reflow, or additional significant adverse events were recorded[55].
Atherectomy
Originally, atherectomy was born as a bail-out strategy for debulking severely calcified un-dilatable stenoses; nowadays, it represents a primary method of lesion preparation by plaque modification[39]. This technology allows less procedural and fluoroscopy times, less contrast volume, and less use of predilatation balloon catheters compared to the bail-out approach[56]. Nowadays, atherectomy techniques include either rotational or orbital atherectomy[39].
Rotational atherectomy
Rotational atherectomy (RA) was born 30 years ago mainly to realize mechanical debulking of atherosclerotic plaque[46] as a stand-alone tool, but it failed to demonstrate superiority over conventional PCI. Nowadays, RA plays a complementary role to PCI and stenting for the preparation of heavily calcified or severely fibrotic lesions that cannot be crossed by a balloon or be adequately dilated.
RA produces differential cutting that permits the mechanical ablation of inelastic fibrocalcific plaques, sparing adjacent normal vessel walls, leading to a change in vessel compliance and increasing the luminal area to facilitate device delivery[57]. OCT after RA has revealed that atherectomy produces a superficial concave groove within both calcified and noncalcified tissue with a trivial effect on the lumen area, and it can create a channel to facilitate stent delivery[58]. A recent single-center, retrospective observational study has shown that by defining the indication and endpoint of plaque modification by RA based on the thickness of calcium, OCT-guided PCI was correlated with significantly higher stent expansion compared with IVUS-guided PCI[59]. RA results in lesion modification with fewer dissections compared to POBA.
RA consists of a 6 or 7 Fr compatible over-the-wire (OTW) system that can be used through radial or femoral access; it includes a rotatory abrasive surface made up of 2,000-3,000 diamonds crystals-encrusted elliptical burr passed over a 0.009″ guidewire with a 0.014″ radiopaque platinum distal tip (Floppy or Extra-support RotaWire). Burr is available in the following sizes: 1.25, 1.5, 1.75, 2.0, 2.15, 2.25, 2.38, and 2.5 mm; it rotates at rates of ~150,000-200,000 revolutions per minute (rpm) and moves on a helical driveshaft. The DyneGlide system permits burr retrieval at a controlled speed rotation of 60,000-90,000 rpm. The abraded plaque is reduced in micro-particles (5-10 µm diameter) that embolize distally through the coronary microcirculation and are phagocytosed by the reticule-endothelial cells. The introduction of the ROTAPRO system (Boston Scientific, Boston, Massachusetts, USA) in 2018 has simplified the controls by upgrading the console and the advancer while preserving the prior burr and drive shaft equipment, resulting in a less challenging procedure. Optimal technique to avoid complications suggests:
- Sizing the burr: artery ratio as 0.5-0.7. Burr: artery ratio > 0.7 had no benefit over PTCA; burr: artery ratio < 0.7 achieved comparable fast lumen enlargement and late vessel revascularization, and it was associated with fewer angiographic complications;
- Rotational speed between 150,000 and 180,000 rpm: very high rotational speeds have been reported to increase distal embolization and procedural myocardial infarction; on the other hand, rotational speed greater than 60,000 rpm is needed to overcome frictional forces and facilitate the passage and retrieval of the burr;
- Use of a pecking motion to allow for frequent intermittent blood flow through the ablated segment, improving wash-out of the debris and reducing heat generation and vessel spasm;
- Limiting ablations to < 20 s, preventing decelerations > 5,000 rpm during ablation, and never stopping the rotations in the lesion.
Initial reports conducted in the DES era that investigated the procedural success of RA were retrospective, non-randomized, observational studies. The ROTAXUS (Rotational Atherectomy Prior to Taxus Stent Treatment for Complex Native Coronary Artery Disease) study was the primary randomized controlled trial that randomized 240 patients to RA or conventional PCI in moderate to heavy CAC. It showed that the RA group was superior to the conventional PCI group for acute procedural success and acute luminal gain but also had significantly higher rates of late luminal loss at nine months[60]. The PREPARE-CALC study, published in 2018, randomized 200 patients with demonstrated myocardial ischemia and heavily calcified plaques to treatment with modified balloons (MB) or RA. It confirmed procedural success in the RA group with no more late lumen loss and similar clinical outcomes at 9 months[61]; results at two years showed that the rates of target vessel failure (TVF) and Major adverse cardiovascular events (MACE) were not significantly different and suggested that RA might be more effective in reducing TVF in long lesions. In contrast, MB appears to be more effective in short ones[62].
Historical contraindications for RA were occlusions in which wiring is impossible, lesions in the last patent vessel in patients with left ventricular dysfunction, lesions in saphenous vein grafts, the presence of thrombus, the presence of major dissection; nowadays, thanks to the operator’s experience, the availability of tools for hemodynamic support and the safety of the system, some of the contraindications were overcome.
Periprocedural complications include cardiac tamponade (0.64%), dissection, perforation, acute vessel closure, side branch loss, slow flow/no-reflow, vasospasm, and burr/wire entrapment[63-65]. Choosing smaller burr sizes reduces angiographic complications without compromising procedural success; Slow flow or no-reflow occurs secondary to distal embolization as well as vasospasm; it may be prevented using an infusion of vasodilator flush cocktail and antiplatelet drugs and should be treated with the delivery of drugs via a microcatheter, using nitroglycerine, adenosine, verapamil, and nicorandil; intravenous boluses of phenylephrine can be used to maintain systolic blood pressure, while reflex bradycardia may require pacing. Sakakura et al. have demonstrated that the occurrence of slow flow did not differ after low-speed RA and high-speed RA[66]; a recent retrospective, single-center trial conducted by Jinnouchi et al. has shown that in lesions treated with RA, the maximum number of reverberations, the lesion length and the arc of calcification at minimal lumen area (MLA) observed at IVUS examination, were associated with slow flow[67]. Burr entrapment may occur in case of aggressive advancement of the burr through very eccentric and extremely calcified lesions. An accurate technique and a smaller burr can avoid this complication. Attempts to restart the burr should be avoided; most times, stable and controlled traction on the drive shaft will release the burr; sometimes, a second arterial access site is needed, and the entrapped burr may be retrieved by balloon angioplasty, advancing a second guide wire (through a second guiding catheter) beyond the entrapped burr. In rare cases, surgical removal of the burr may be necessary[68]. Wire entrapment with or without distal entrapment of the wire may be caused by a fracture in the transition zone of the wire. It may be solved by advancing up the point of entrapment to a microcatheter or an OTW balloon and exerting a steady pull-back; if this does not work, breaking the guidewire and leaving the residual wire in site without significant complications is possible. When treating a bifurcation, it is essential to remember that wiring a side branch for side-branch protection during RA is not possible, but Medda et al. first described the use of a “double-guiding catheter technique” to protect the side branch during RA for the treatment of Left Main bifurcation[69].
In the setting of aorto-ostial stenosis, it is suggested to choose a larger guiding catheter to start RA within the catheter and to keep it coaxial to avoid burr lodging[70].
Orbital atherectomy
The Diamondback 360° Coronary Orbital Atherectomy (OA) System (Cardiovascular Systems Inc., St. Paul, MN, USA) is a percutaneous technology designated to modify heavily calcified coronary plaques and enable stent delivery and expansion[39,71-73]. OA was authorized in the USA in 2013 and received a CE mark in January 2021. OCT has revealed that OA produces grooves within the surface of the vessel wall (both calcified and noncalcified) that are deeper than RA with consequently more effect on lipid-rich lesions; like RA, it has a restricted effect on deeper calcification and lumen area, but studies have demonstrated that OA can fracture calcified lesions, thereby increasing lesion compliance and facilitating stent expansion; in this setting, OCT can be used to estimate the total ablation volume[58,74].
OA consists of a 6 Fr compatible system that includes a 1.25 mm diameter diamond-coated crown, which orbits over a specific stainless-steel wire (0.0012″ ViperWire Advance) in an elliptical route, applying centrifugal energy on the arterial wall able to pulverize the engaged calcified and fibrotic tissue, while softer tissue flexes away from the crown[75]. The ablative component is placed alongside the coil, consisting of three helically wound wires that can be constricted by applying pressure. The operator can regulate the ablation intensity by raising rotational speed, translating to a larger rotation orbit. To obtain the best performance, it is recommended to have a continuous action (because the risk of crown entrapment is minor) at low or elevated speeds (80,000 or 120,000 rpm, respectively), do not exceed 30 s for each run, a fast constant infusion of the proprietary lubricant ViperSlide flush and application of retrograde ablation to prevent device entrapment and to reach a total ablation[76]. GlideAssist Mode (5,000 rpm spin) can be activated to facilitate device insertion/removal. Potential benefits over RA consist of bidirectional therapy of an atherosclerotic plaque, including the treatment of ostial lesions, the possibility to treat different vessel diameters with a single-size burr and potentially more profound calcification, the reduction of heat injury, decreased rate of transient heart block and no-reflow thanks to the continuous blood flow maintained during ablation and the production of microparticulate debris averaging less than 2 mm in size[58,74,77]. In addition, Panchal et al. recently reported the use of a microcatheter with a single guide catheter to protect the side branch during OA for the treatment of heavily calcified bifurcation[78].
In the setting of aorto-ostial stenosis, it is suggested to perform OA retrograde if the device crosses the lesion, and to keep the guiding catheter coaxial to allow engagement if the system does not cross at first the calcification.
Nowadays, there are no studies that have compared OA and RA ablation ability and capability to treat tight calcified stenosis. An additional OA device that includes a tapered micro crown system incorporating a diamond-coated tip in addition to the classic crown, that works at reduced speed (50,000 or 80,000 rpm), has been approved in the USA and Japan; it was evaluated in 100 patients in the Coronary Orbital Atherectomy System Study (COAST) trial for the use in tighter lesions[79,80].
The ORBIT I trial (Safety and feasibility of orbital atherectomy for the treatment of calcified coronary lesions)[81] and the ORBIT II trial (Evaluate the Safety and Efficacy of OAS in Treating Severely Calcified Coronary Lesions)[82] were two single-arm studies that established the safety and the success of OA. The results were confirmed by a real-world multicentre registry that also documented fewer angiographic complications and MACE[75,83]. A recent small prospective single-center study conducted by Yap et al. has demonstrated the feasibility and safety of OA in managing calcified coronary stenosis and the critical role of IVUS and OCT in guiding the whole procedure[84]. The Nationwide Readmissions Database in the USA has recently shown that intravascular imaging application during PCI with OA for CAC lesions seems to be related to lower in-hospital mortality at index admission[85]. A prospective, multicentre, randomized trial is ongoing comparing the ordinary utilization of the Diamondback 360° Coronary OAS with conventional balloon angioplasty before DES implantation in heavy CAC (ECLIPSE Trial). It includes an OCT substudy assessing the final minimal stent area (MSA)[86].
Excimer laser atherectomy
The Excimer laser coronary atherectomy (ELCA) (Spectranetics, Colorado Springs, Colorado, USA) uses a pulsed excimer or holmium laser power to create ultraviolet pulses at 308 nm that can enlarge hardy stenosis via a photochemical system (carbon bonds destruction in the vessel wall), a photothermal effect (cellular destruction by intracellular water heating), and a photomechanical mechanism (explosion of bubbles generated at the catheter tip)[87]. The microparticles (< 10 m) embolize distally through the coronary microcirculation and are phagocytosed by the reticule-endothelial system. ELCA can modify the atherosclerotic plaque at the level of the intima with the preservation of media and adventitia. The ELCA catheter is available in 0.9, 1.4 mm (6 Fr. Compatible), and 1.7- and 2.0-mm (7 Fr. compatible) diameters; it has a monorail system that is compatible with any 0.014″ coronary guidewire; consequently, it can be useful when wire exchange is not possible. To minimize the risk of dissection, it is essential to remember to use intracoronary saline infusion during the ELCA[88].
Its primary application currently is for uncrossable or un-dilatable lesions with conventional balloons[89], facilitating device delivery.
Furthermore, it has been successfully utilized to modify the lesion’s proximal cap of a CTO in the setting of ISR[90], in the treatment of complex coronary lesions with moderate calcification before DES implantation[91] and in the rare case of ISR due to stent underexpansion for the presence of severe calcifications in the vessel wall[92]. The application of LA in difficult un-dilatable stenosis, mostly due to the presence of calcification, has been assessed in several minor retrospective registries[93,94]. Small case series have shown the creation of dissection planes at OCT images exerted by ELCA in calcified lesions[95]. Another minor case series revealed intimal disruption and many limited zones of calcium fracture[96]. The LEONARDO study demonstrated great procedural success (93.7%) and low procedural complications in treating balloon-resistant calcified plaques just with ELCA, without dissections, perforations, or slow flow[97]. A recent multi-center registry has demonstrated the safety and efficacy of ELCA but showed its failure in severe calcifications, with the need for RA as a bail-out technique[98]. In addition, ELCA seems to be effective in the treatment of ISR due to underexpanded stents because of severely calcified lesions, even if there are only small case series to support this use. Complications include overheating, which could cause vascular injury and may be due to the stop of the saline flush or unintentional contrast injection. ELCA has no absolute contraindications, and only a relative contraindication, which is the subintimal passage of the guidewire, like techniques used for CTO PCI.
Intravascular lithotripsy
Intravascular lithotripsy (IVL) applies pulsatile mechanical energy to disrupt superficial and profound calcifications with the same mechanism utilized for nephrolithiasis.
The Shockwave Medical coronary IVL catheter (Medical INC, Fremont, CA, USA) is a 0.014″ guidewire compatible angioplasty catheter, with a 12-mm-long fluid-filled balloon that includes two lithotripsy emitters into the shaft[99]. The IVL balloon catheter is projected for single use and is made of a 6 Fr compatible monorail system with a dual port hub, one connected to the balloon indeflator and the other connected to a transportable, rechargeable, nonsterile battery-powered generator. The lithotripsy emitters receive energy when the balloon is inflated to 4-6 atm. Electrical power is able to generate steam bubbles that expand and collapse, creating brief (~1 millisecond) acoustic force pulses/sonic tension waves that produce ~50 atm of direct pressure to selectively disrupt the calcium[100,101]. These sonic pressure waves are released circumferentially and transmurally in the arterial wall, resulting in calcium micro-fractures thanks to brief shear mechanisms[102]. The coronary IVL balloon is offered in 2.5-, 3.0-, 3.5-, and 4.0-mm diameters and 12-mm lengths. The working length of the fast exchange catheter is 138 cm and it delivers up to 80 total IVL pulses at a speed of one pulse per second (8 sequences of 10 impulses). A minimum of 20 pulses is delivered in the calcified lesion, followed by transient deflation to permit distal perfusion.
IVL has been extensively employed in the management of peripheral artery disease as a stand-alone tool and also to facilitate stent deployment. Single-arm, non-randomized clinical trials have assessed the safety and efficacy of IVL in the setting of CAD, in addition to coronary stenting[100,102,103]. The Disrupt CAD I was a prospective, multi-center, single-arm trial that included 60 patients with heavy calcifications of 21 mm average length and reached a 98,3% device success and a 92% residual stenosis < 30%[100]. MACE (composite of cardiac death, non-Q-wave MI, and target vessel revascularization (TVR) through 30 days) comprised only three patients (5%) with non-Q MI. The Disrupt CAD II was a prospective, multi-center trial that evaluated IVL treatment in 120 patients with heavy CAC of 25.7 mm in length. Acute lumen gain after only IVL was 0.83 ± 0.47 mm with residual stenosis of 32.7% ± 10.4%. Only 7.6% of patients experienced MACE at 30 days[103]. The OCT substudy conducted in 47 patients explained the principal mechanism of action of IVL by identifying calcium fractures in 78.7% of lesions[103]. The Disrupt CAD III was a prospective, single-arm, multi-center trial intended for regulatory authorization of coronary IVL that included 431 patients at 47 places in four countries; it demonstrated the safety and efficacy of the device. OCT substudy revealed multi-plane and longitudinal intraplaque fractures after IVL in 67.4% of CAC [Figure 4C]. MSA was
The advantages of IVL consist of the facility of utilization with a fast learning curve, absence of distal embolization/slow flow-no-reflow, and less risk of barotrauma-related dissections and rupture thanks to the lower-pressure balloon inflations[99]. Additionally, wiring a side branch for side-branch protection is possible, without the risk of wire entrapment or damage that may happen with RA or OA[99]. Sometimes, IVL catheter cannot cross a calcified lesion because of the great tightness; in these cases, it is suggested to pre-dilate with conventional angioplasty balloons or to use RA to facilitate device delivery. Recently, the first use of IVL with robotically assisted PCI has been reported[107]. A recent systematic review conducted by Sheikh et al. revealed that the use of IVL with intravascular imaging has transformed the therapy of heavily calcified, un-dilatable coronary lesions and is likely to replace the conventional methods of treating these complex lesions[108]. Furthermore, IVL may be useful in the treatment of undilatable ISR due to stent underexpansion in the setting of heavily calcified lesions, as described by many case reports[109].
SUPERFICIAL, DEEP CALCIFICATIONS AND CALCIFIED NODULE: DOES ONE DEVICE FIT ALL?
The chondrocyte-like cells and the manifestation of inflammatory elements, together with cells that promote bone deposition destroying collagen fibers, are responsible for the dysmorphic calcium precipitation that causes the atherosclerotic calcification development[110].
Calcification appears to precede inflammation, contributing to its evolution, with the two activities coexisting and promoting each other[13]. Superficial calcifications are located in the intima, whereas deep calcifications are in the media. IVUS better identifies deep CAC, but it cannot detect a deep calcific deposit behind a superficial calcific deposit and cannot estimate the calcium thickness. Atheroablative technologies (RA or OA) tend to ablate the luminal surface. They thus are indicated for treating extensive superficial calcifications[14], whereas IVL is preferred for deep calcification, thanks to its ability to create calcium fractures.
Eruptive CN is a form of CAC responsible for 2%-7% of acute coronary events. In a recent study conducted on patients with acute coronary syndrome (ACS) who had undergone pre-intervention OCT, three calcified culprit plaques were recognized: eruptive CN, superficial calcific sheet, and calcified protrusion[111]. Nodular calcification [Figure 4B] histologically consists of zones of calcification of variable dimensions, frequently accompanied by fibrin with a thick, integral fibrous cap. In eruptive CN, the fibrous cap is disrupted, with an overlying platelet/fibrin thrombus and no endothelium cells. These nodules are usually eccentric and can also disrupt the medial wall[112]. CN has been reported to be associated with stent failure, including ISR and stent thrombosis[113-115]. Nagata et al. have recently shown that the combined presence at OCT of eruptive CN and medial dissection with calcified flaps within the DES-implanted segment is correlated with a greater incidence of cumulative device-oriented composite endpoints (DoCE) after DES implantation[116]. The treatment of CN has not been established; however, RA is the preferred device in this setting because it can ablate the calcified structure within the CN, facilitating stent expansion. However, in a recent retrospective study, CN was associated with increased DoCE in patients treated with RA[117].
Characterization of calcium lesions is essential to choosing the adequate device; nowadays, several algorithms suggest which tool to treat CAC based on calcium characteristics [Figure 6]. However, in some cases, a single system to modify calcium is insufficient, because every device has advantages and disadvantages [Table 1]. Thanks to the growing operator’s confidence and expertise, more than one device is increasingly used to treat heavy coronary calcification in a synergistic combination that allows optimal lesion preparation to facilitate stent delivery.
Engineering and clinical characteristics with pros and cons of several debulking devices utilized in coronary intervention
Characteristics | Cutting/scoring/high-pressure balloon | Rotational atherectomy | Orbital atherectomy | Intravascular litotripsy | Laser |
Guidewire | 0.014″ Preferred wire | Rotawire drive - 0.09″ body and 0.014″ spring tip | Viper wire - 0.012″ body and 0.014″ spring tip | 0.014″ Preferred wire | 0.014″ Preferred wire |
Effect of wire bias on calcium modification | Independent, circumferential calcium modification | Wire-bias dependent during push ablation | Less wire-bias dependent Push ablation - more at the outer vessel side Pull ablation - more at the inner vessel side | Independent, circumferential calcium modification | Dependent on vessel angulation (since ultraviolet light does not bend) with circumferential calcium modification |
Side branch protection | No interaction with side branch wire | Side branch wire must be removed during atherectomy (possible protection with coiled shaft microcatheter with double-guiding catheter technique) | Side branch wire must be removed during atherectomy (possible protection with coiled shaft microcatheter with double-guiding catheter technique) | No interaction with side branch wire | No interaction with side branch wire |
Distal embolization | None | Releases debris and microparticles | Releases debris and microparticles | None | None |
Perforation | Low (< 1%) | Up to 1.5% | Up to 1.8% | Low (< 1%) | Up to 1.8% |
Bradyarrhythmia | Not necessary | Temporary pacemaker suggested in dominant coronary artery | Temporary pacemaker suggested in dominant coronary artery | Not necessary | Not necessary |
Plaque ablation | No plaque ablation | Dependent on the selected burr size | Dependent on the minimal lumen area | No plaque ablation | Dependent on the selected laser catheter size and type of medium interface utilized (saline, blood, contrast) |
Effect on intimal (superficial) and media (deep) calcification | Ablates superficial and deep calcium | Ablates superficial calcium | Ablates superficial and deep calcium | Ablates superficial and deep calcium | Different actions on superficial and deep calcium (light pulsed, acoustic pressure waves, cavitation bubbles) |
The PREPARE-CALC-COMBO study evaluated the safety and efficacy of lesion preparation using RA with successive CB angioplasty (Rota‐Cut). It revealed that Rota‐Cut strategy lead to greater acute lumen gain and higher MSA compared with RA or CB alone but was not associated with better stent expansion; TVF was comparable and low at 9 months[118]; a randomized clinical trial conducted in China that enrolled 120 patients treated with IVUS-guided Rota-Cut angioplasty showed similar results[119].
The Rota-Shock (Rota-tripsy) strategy to treat both luminal and abluminal calcification with RA and IVL was first evaluated in a small case series in USA[120] and in a small retrospective study[121]; a recent study involved 21 patients with de novo calcified lesions (12) and ISR (9) treated with Rota-shock strategy demonstrated procedural success in 20 cases, with IVL balloon crossing the lesion in all cases following RA[122]. A recent retrospective analysis of 25 patients treated with rota-shock or orbital-shock, almost IVUS-guided, has revealed the efficacy of this strategy and the relevant role of IVUS in guiding the procedure[123]. Combined use of IVL with ELCA has been described in one case report, supporting this strategy when the use of RA is discouraged[124]. Recently, a case of a combination of OA use followed by RA has been described by Yamamoto et al.[125].
CONCLUSION
The percutaneous management of complex coronary artery calcific disease is becoming more frequent, mainly related to an increasingly older population of patients with more extensive coronary disease and multiple comorbidities. Therefore, the interventional cardiologist must overcome the historical challenge of CAC, becoming more confident in new technologies to offer patients a safe and effective percutaneous solution. Recent developments in intravascular imaging and systems that modify calcium allow for a tailored and dynamic percutaneous treatment to achieve high success in PCI and the best clinical outcome. In this scenario, intracoronary imaging could also have a key role in evaluating the effect of new devices on coronary vessels: the future is just around the corner.
DECLARATIONS
Authors’ contributions
Made substantial contributions to the conception and design of the study and performed data analysis and interpretation: Russo D, Massaro G, Sangiorgi GM, Chiricolo G, Benedetto D
Performed data acquisition, as well as providing administrative, technical, and material support: Russo G, Pescetelli I, Canova P, Fiocca L, Romano M, Lettieri C
Availability of data and materials
Not applicable.
Financial support and sponsorship
None.
Conflicts of interest
All authors declared that there are no conflicts of interest.
Ethical approval and consent to participate
Not applicable.
Consent for publication
Not applicable.
Copyright
© The Author(s) 2024.
REFERENCES
1. Bourantas CV, Zhang YJ, Garg S, et al. Prognostic implications of coronary calcification in patients with obstructive coronary artery disease treated by percutaneous coronary intervention: a patient-level pooled analysis of 7 contemporary stent trials. Heart 2014;100:1158-64.
2. Abdelrahman KM, Chen MY, Dey AK, et al. Coronary computed tomography angiography from clinical uses to emerging technologies: JACC state-of-the-art review. J Am Coll Cardiol 2020;76:1226-43.
3. Newby DE, Adamson PD, Berry C, et al. Coronary CT angiography and 5-year risk of myocardial infarction. N Engl J Med 2018;379:924-33.
4. Busse A, Cantré D, Beller E, et al. Cardiac CT: why, when, and how: update 2019. Herz-CT: warum, wann und wie. Radiologe 2019;59:1-9.
5. Budoff MJ, Young R, Burke G, et al. Ten-year association of coronary artery calcium with atherosclerotic cardiovascular disease (ASCVD) events: the multi-ethnic study of atherosclerosis (MESA). Eur Heart J 2018;39:2401-8.
6. Willemink MJ, Leiner T, Maurovich-Horvat P. Cardiac CT imaging of plaque vulnerability: hype or hope? Curr Cardiol Rep 2016;18:37.
7. Irkle A, Vesey AT, Lewis DY, et al. Identifying active vascular microcalcification by 18F-sodium fluoride positron emission tomography. Nat Commun 2015;6:7495.
8. Dweck MR, Chow MWL, Joshi NV, et al. Coronary arterial 18F-sodium fluoride uptake: a novel marker of plaque biology. J Am Coll Cardiol 2012;59:1539-48.
9. Rosa GM, Bauckneht M, Masoero G, et al. The vulnerable coronary plaque: update on imaging technologies. Thromb Haemost 2013;110:706-22.
10. Wang Y, Osborne MT, Tung B, Li M, Li Y. Imaging cardiovascular calcification. J Am Heart Assoc 2018;7:e008564.
11. Sheahan M, Ma X, Paik D, et al. Atherosclerotic plaque tissue: noninvasive quantitative assessment of characteristics with software-aided measurements from conventional CT angiography. Radiology 2018;286:622-31.
12. Qiao J, Li S, Yang H, et al. Subtraction improves the accuracy of coronary CT angiography in patients with severe calcifications in identifying moderate and severe stenosis: a multicenter study. Acad Radiol 2023;30:2801-10.
13. Sun Z, Ng CKC. Artificial intelligence (enhanced super-resolution generative adversarial network) for calcium deblooming in coronary computed tomography angiography: a feasibility study. Diagnostics 2022;12:991.
14. Fink N, Zsarnoczay E, Schoepf UJ, et al. Photon counting detector CT-based virtual noniodine reconstruction algorithm for in vitro and in vivo coronary artery calcium scoring: impact of virtual monoenergetic and quantum iterative reconstructions. Invest Radiol 2023;58:673-80.
15. Andreini D, Collet C, Leipsic J, et al. Pre-procedural planning of coronary revascularization by cardiac computed tomography. J Cardiovasc Comput Tomogr 2022;16:558-72.
16. Kurogi K, Ishii M, Nagatomo T, et al. Mean density of computed tomography for predicting rotational atherectomy during percutaneous coronary intervention. J Cardiovasc Comput Tomogr 2023;17:120-9.
17. Simsek B, Jaffer FA, Kostantinis S, et al. Preprocedural coronary computed tomography angiography in chronic total occlusion percutaneous coronary intervention: insights from the PROGRESS-CTO registry. Int J Cardiol 2022;367:20-5.
18. Yu CW, Lee HJ, Suh J, et al. Coronary computed tomography angiography predicts guidewire crossing and success of percutaneous intervention for chronic total occlusion: Korean multicenter CTO CT registry score as a tool for assessing difficulty in chronic total occlusion percutaneous coronary intervention. Circ Cardiovasc Imaging 2017;10:e005800.
19. Available from: https://www.eurocto.eu/slides/en-GB/526/session-6-ct-guided-cto-pci [Last accessed on 26 Feb 2024].
20. Panuccio G, Tonini G, Erbay A, et al. Impact of coronary computed tomography angiography derived full Moon calcifications in CTO-PCI. Eur Heart J 2023;44:ehad655.2203.
21. Dini C, Nardi G, Ristalli F, Mattesini A, Hamiti B, Di Mario C. Contemporary approach to heavily calcified coronary lesions. Interv Cardiol 2019;14:154-63.
22. Hennessey B, Pareek N, Macaya F, et al. Contemporary percutaneous management of coronary calcification: current status and future directions. Open Heart 2023;10:e002182.
23. Wang X, Matsumura M, Mintz GS, et al. In vivo calcium detection by comparing optical coherence tomography, intravascular ultrasound, and angiography. JACC Cardiovasc Imaging 2017;10:869-79.
24. Zaffino P, Spadea MF, Indolfi C, De Rosa S. CoroFinder: a new tool for real time detection and tracking of coronary arteries in contrast-free cine-angiography. J Pers Med 2022;12:411.
25. Piayda K, Kleinebrecht L, Afzal S, et al. Dynamic coronary roadmapping during percutaneous coronary intervention: a feasibility study. Eur J Med Res 2018;23:36.
26. Friedrich GJ, Moes NY, Mühlberger VA, et al. Detection of intralesional calcium by intracoronary ultrasound depends on the histologic pattern. Am Heart J 1994;128:435-41.
27. Kawasaki M, Bouma BE, Bressner J, et al. Diagnostic accuracy of optical coherence tomography and integrated backscatter intravascular ultrasound images for tissue characterization of human coronary plaques. J Am Coll Cardiol 2006;48:81-8.
28. Witzenbichler B, Maehara A, Weisz G, et al. Relationship between intravascular ultrasound guidance and clinical outcomes after drug-eluting stents: the assessment of dual antiplatelet therapy with drug-eluting stents (ADAPT-DES) study. Circulation 2014;129:463-70.
29. Choi KH, Song YB, Lee JM, et al. Impact of intravascular ultrasound-guided percutaneous coronary intervention on long-term clinical outcomes in patients undergoing complex procedures. JACC Cardiovasc Interv 2019;12:607-20.
30. Zhang M, Matsumura M, Usui E, et al. Intravascular ultrasound-derived calcium score to predict stent expansion in severely calcified lesions. Circ Cardiovasc Interv 2021;14:e010296.
31. You W, Zhang HL, Xu T, et al. Preliminary study of the significance of reverberation by IVUS detection for patients with severe calcified lesions. Int J Cardiovasc Imaging 2023;39:667-76.
32. Räber L, Mintz GS, Koskinas KC, et al. Clinical use of intracoronary imaging. Part 1: guidance and optimization of coronary interventions. An expert consensus document of the European Association of Percutaneous Cardiovascular Interventions. Eur Heart J 2018;39:3281-300.
33. Hennessey B, Vera-Urquiza R, Mejía-Rentería H, Gonzalo N, Escaned J. Contemporary use of coronary computed tomography angiography in the planning of percutaneous coronary intervention. Int J Cardiovasc Imaging 2020;36:2441-59.
34. Kobayashi Y, Okura H, Kume T, et al. Impact of target lesion coronary calcification on stent expansion. Circ J 2014;78:2209-14.
35. Fujino A, Mintz GS, Lee T, et al. Predictors of calcium fracture derived from balloon angioplasty and its effect on stent expansion assessed by optical coherence tomography. JACC Cardiovasc Interv 2018;11:1015-7.
36. Maejima N, Hibi K, Saka K, et al. Relationship between thickness of calcium on optical coherence tomography and crack formation after balloon dilatation in calcified plaque requiring rotational atherectomy. Circ J 2016;80:1413-9.
37. Tearney GJ, Regar E, Akasaka T, et al. Consensus standards for acquisition, measurement, and reporting of intravascular optical coherence tomography studies: a report from the international working group for intravascular optical coherence tomography standardization and validation. J Am Coll Cardiol 2012;59:1058-72.
38. Mario C, Ramasami N. Techniques to enhance guide catheter support. Catheter Cardiovasc Interv 2008;72:505-12.
39. Kassimis G, Raina T, Kontogiannis N, et al. How should we treat heavily calcified coronary artery disease in contemporary practice? From atherectomy to intravascular lithotripsy. Cardiovasc Revasc Med 2019;20:1172-83.
40. Costopoulos C, Naganuma T, Colombo A. Tools and techniques clinical: percutaneous intervention of calcific coronary lesions. EuroIntervention 2014;9:1124-6.
41. Okura H, Hayase M, Shimodozono S, et al. Mechanisms of acute lumen gain following cutting balloon angioplasty in calcified and noncalcified lesions: an intravascular ultrasound study. Catheter Cardiovasc Interv 2002;57:429-36.
42. Tang Z, Bai J, Su SP, et al. Cutting-balloon angioplasty before drug-eluting stent implantation for the treatment of severely calcified coronary lesions. J Geriatr Cardiol 2014;11:44-9.
43. Mangieri A, Nerla R, Castriota F, et al. Cutting balloon to optimize predilation for stent implantation: the COPS randomized trial. Catheter Cardiovasc Interv 2023;101:798-805.
44. Ikenaga H, Kurisu S, Kihara Y. Optical coherence tomography findings after scoring balloon dilatation. Rev Esp Cardiol 2015;68:1022.
45. Benezet J, Díaz de la Llera LS, Cubero JM, Villa M, Fernández-Quero M, Sánchez-González A. Drug-eluting stents following rotational atherectomy for heavily calcified coronary lesions: long-term clinical outcomes. J Invasive Cardiol 2011;23:28-32.
46. Fourrier JL, Bertrand ME, Auth DC, Lablanche JM, Gommeaux A, Brunetaud JM. Percutaneous coronary rotational angioplasty in humans: preliminary report. J Am Coll Cardiol 1989;14:1278-82.
47. Abdel-Wahab M, Richardt G, Büttner JH, et al. High-speed rotational atherectomy before paclitaxel-eluting stent implantation in complex calcified coronary lesions: the randomized ROTAXUS (rotational atherectomy prior to taxus stent treatment for complex native coronary artery disease) trial. JACC Cardiovasc Interv 2013;6:10-9.
48. de Ribamar Costa J Jr, Mintz GS, Carlier SG, et al. Nonrandomized comparison of coronary stenting under intravascular ultrasound guidance of direct stenting without predilation versus conventional predilation with a semi-compliant balloon versus predilation with a new scoring balloon. Am J Cardiol 2007;100:812-7.
49. Otsuka Y, Koyama T, Imoto Y, et al. Prolonged inflation technique using a scoring balloon for severe calcified lesion. Int Heart J 2017;58:982-7.
50. Dayco JS, Osman H, Almas T, et al. Major Complications and failure modes of the angiosculpt scoring balloon catheter: analysis of the MAUDE database. Curr Probl Cardiol 2023;48:101557.
51. Secco GG, Ghione M, Mattesini A, et al. Very high-pressure dilatation for undilatable coronary lesions: indications and results with a new dedicated balloon. EuroIntervention 2016;12:359-65.
52. Secco GG, Buettner A, Parisi R, et al. Clinical experience with very high-pressure dilatation for resistant coronary lesions. Cardiovasc Revasc Med 2019;20:1083-7.
53. Rheude T, Rai H, Richardt G, et al. Super high-pressure balloon versus scoring balloon to prepare severely calcified coronary lesions: the ISAR-CALC randomised trial. EuroIntervention 2021;17:481-8.
54. Scalamogna M, Abdel-Wahab M, Mashayekhi K, et al. Randomized ComparIson of strategies to PrepAre SeveRely CALCified coronary lesions 2: design and rationale of the ISAR-CALC 2 trial. Cardiovasc Revasc Med 2023;49:22-7.
55. Pinilla-Echeverri N, Bossard M, Hillani A, et al. Treatment of calcified lesions using a dedicated super-high pressure balloon: multicenter optical coherence tomography registry. Cardiovasc Revasc Med 2023;52:49-58.
56. Kawamoto H, Latib A, Ruparelia N, et al. In-hospital and midterm clinical outcomes of rotational atherectomy followed by stent implantation: the ROTATE multicentre registry. EuroIntervention 2016;12:1448-56.
57. Neumann FJ, Sousa-Uva M, Ahlsson A, et al. 2018 ESC/EACTS guidelines on myocardial revascularization. Eur Heart J 2019;40:87-165.
58. Kini AS, Vengrenyuk Y, Pena J, et al. Optical coherence tomography assessment of the mechanistic effects of rotational and orbital atherectomy in severely calcified coronary lesions. Catheter Cardiovasc Interv 2015;86:1024-32.
59. Kurogi K, Ishii M, Ikebe S, et al. Optical coherence tomography-versus intravascular ultrasound-guided stent expansion in calcified lesions. Cardiovasc Interv Ther 2022;37:312-23.
60. de Waha S, Allali A, Büttner HJ, et al. Rotational atherectomy before paclitaxel-eluting stent implantation in complex calcified coronary lesions: two-year clinical outcome of the randomized ROTAXUS trial. Catheter Cardiovasc Interv 2016;87:691-700.
61. Abdel-Wahab M, Toelg R, Byrne RA, et al. High-speed rotational atherectomy versus modified balloons prior to drug-eluting stent implantation in severely calcified coronary lesions. Circ Cardiovasc Interv 2018;11:e007415.
62. Allali A, Toelg R, Abdel-Wahab M, et al. Combined rotational atherectomy and cutting balloon angioplasty prior to drug-eluting stent implantation in severely calcified coronary lesions: the PREPARE-CALC-COMBO study. Catheter Cardiovasc Interv 2022;100:979-89.
63. Furuichi S, Sangiorgi GM, Godino C, et al. Rotational atherectomy followed by drug-eluting stent implantation in calcified coronary lesions. EuroIntervention 2009;5:370-4.
64. Vaquerizo B, Serra A, Miranda F, et al. Aggressive plaque modification with rotational atherectomy and/or cutting balloon before drug-eluting stent implantation for the treatment of calcified coronary lesions. J Interv Cardiol 2010;23:240-8.
65. Naito R, Sakakura K, Wada H, et al. Comparison of long-term clinical outcomes between sirolimus-eluting stents and paclitaxel-eluting stents following rotational atherectomy. Int Heart J 2012;53:149-53.
66. Sakakura K, Funayama H, Taniguchi Y, et al. The incidence of slow flow after rotational atherectomy of calcified coronary arteries: a randomized study of low speed versus high speed. Catheter Cardiovasc Interv 2017;89:832-40.
67. Jinnouchi H, Sakakura K, Taniguchi Y, et al. Intravascular ultrasound-factors associated with slow flow following rotational atherectomy in heavily calcified coronary artery. Sci Rep 2022;12:5674.
68. Sulimov DS, Abdel-Wahab M, Toelg R, Kassner G, Geist V, Richardt G. Stuck rotablator: the nightmare of rotational atherectomy. EuroIntervention 2013;9:251-8.
69. Medda M, Casilli F, Bande M, Tespili M. Protected rotational atherectomy with double-guiding catheter technique for unprotected distal left main. J Am Coll Cardiol Interv 2019;12:e27-9.
70. Barbato E, Carrié D, Dardas P, et al. European expert consensus on rotational atherectomy. EuroIntervention 2015;11:30-6.
71. Barbato E, Shlofmitz E, Milkas A, Shlofmitz R, Azzalini L, Colombo A. State of the art: evolving concepts in the treatment of heavily calcified and undilatable coronary stenoses - from debulking to plaque modification, a 40-year-long journey. EuroIntervention 2017;13:696-705.
72. Yamamoto MH, Maehara A, Karimi Galougahi K, et al. Mechanisms of orbital versus rotational atherectomy plaque modification in severely calcified lesions assessed by optical coherence tomography. JACC Cardiovasc Interv 2017;10:2584-6.
73. Shipman JN, Agasthi P. Orbital atherectomy. Treasure Island, FL: StatPearls Publishing. 2023. Available from: http://www.ncbi.nlm.nih.gov/books/NBK563144/ [Last accessed on 26 Feb 2024].
74. Sotomi Y, Cavalcante R, Shlofmitz RA, et al. Quantification by optical coherence tomography imaging of the ablation volume obtained with the orbital atherectomy system in calcified coronary lesions. EuroIntervention 2016;12:1126-34.
75. Parikh K, Chandra P, Choksi N, Khanna P, Chambers J. Safety and feasibility of orbital atherectomy for the treatment of calcified coronary lesions: the ORBIT I trial. Catheter Cardiovasc Interv 2013;81:1134-9.
76. Shlofmitz E, Martinsen BJ, Lee M, et al. Orbital atherectomy for the treatment of severely calcified coronary lesions: evidence, technique, and best practices. Expert Rev Med Devic 2017;14:867-79.
77. Sotomi Y, Shlofmitz RA, Colombo A, Serruys PW, Onuma Y. Patient selection and procedural considerations for coronary orbital atherectomy system. Interv Cardiol 2016;11:33-8.
78. Panchal HB, Nagabandi A, Kirtane AJ, Beohar N. Microcatheter-protected orbital atherectomy for severely calcified bifurcation coronary artery disease with single-guiding catheter technique. JACC Cardiovasc Interv 2020;13:e1-3.
79. Redfors B, Sharma SK, Saito S, et al. Novel micro crown orbital atherectomy for severe lesion calcification: coronary orbital atherectomy system study (COAST). Circ Cardiovasc Interv 2020;13:e008993.
80. Martinsen BJ, Kumar K, Saito S, et al. Japan-USA orbital atherectomy for calcific coronary lesions: COAST study, harmonization by doing proof-of-concept. Cardiovasc Revasc Med 2022;37:112-7.
81. Chambers JW, Feldman RL, Himmelstein SI, et al. Pivotal trial to evaluate the safety and efficacy of the orbital atherectomy system in treating de novo, severely calcified coronary lesions (ORBIT II). JACC Cardiovasc Interv 2014;7:510-8.
82. Généreux P, Lee AC, Kim CY, et al. Orbital atherectomy for treating de novo severely calcified coronary narrowing (1-year results from the pivotal ORBIT II trial). Am J Cardiol 2015;115:1685-90.
83. Bhatt P, Parikh P, Patel A, et al. Long-term safety and performance of the orbital atherectomy system for treating calcified coronary artery lesions: 5-year follow-up in the ORBIT I trial. Cardiovasc Revasc Med 2015;16:213-6.
84. Yap LB, Choy CN, Navin S, Koh KW, Jeyamalar R, Balachandran K. Intravascular imaging-guided treatment of severe coronary artery calcification with orbital atherectomy: a prospective single-centre registry. Med J Malaysia 2023;78:7-13.
85. Sandhyavenu H, Ullah W, Badu I, et al. Outcomes of intravascular imaging in orbital atherectomy; insight from the national readmissions database. Curr Probl Cardiol 2023;48:101475.
86. Généreux P, Kirtane AJ, Kandzari DE, et al. Randomized evaluation of vessel preparation with orbital atherectomy prior to drug-eluting stent implantation in severely calcified coronary artery lesions: design and rationale of the ECLIPSE trial. Am Heart J 2022;249:1-11.
87. Baumbach A, Bittl JA, Fleck E, et al. Acute complications of excimer laser coronary angioplasty: a detailed analysis of multicenter results. J Am Coll Cardiol 1994;23:1305-13.
88. Deckelbaum LI, Natarajan MK, Bittl, JA, et al. Effect of intracoronary saline infusion on dissection during excimer laser coronary angioplasty: a randomized trial. The percutaneous excimer laser coronary angioplasty (PELCA) investigators. J Am Coll Cardiol 1995;26:1264-9.
89. Bilodeau L, Fretz EB, Taeymans Y, Koolen J, Taylor K, Hilton DJ. Novel use of a high-energy excimer laser catheter for calcified and complex coronary artery lesions. Catheter Cardiovasc Interv 2004;62:155-61.
91. Badr S, Ben-Dor I, Dvir D, et al. The state of the excimer laser for coronary intervention in the drug-eluting stent era. Cardiovasc Revasc Med 2013;14:93-8.
92. Alfonso F, Coughlan JJ, Giacoppo D, Kastrati A, Byrne RA. Management of in-stent restenosis. EuroIntervention 2022;18:e103-23.
93. Ben-Dor I, Maluenda G, Pichard AD, et al. The use of excimer laser for complex coronary artery lesions. Cardiovasc Revasc Med 2011;12:69.e1-8.
94. Fretz EB, Smith P, Hilton JD. Initial experience with a low profile, high energy excimer laser catheter for heavily calcified coronary lesion debulking: parameters and results of first seven human case experiences. J Interv Cardiol 2001;14:433-7.
95. Rawlins J, Talwar S, Green M, O’Kane P. Optical coherence tomography following percutaneous coronary intervention with excimer laser coronary atherectomy. Cardiovasc Revasc Med 2014;15:29-34.
96. Gemma D, Galeote García G, Sánchez-Recalde Á. Effects of excimer laser coronary atherectomy assessed by OCT. Rev Esp Cardiol 2017;70:116.
97. Ambrosini V, Sorropago G, Laurenzano E, et al. Early outcome of high energy laser (Excimer) facilitated coronary angioplasty ON hARD and complex calcified and balloOn-resistant coronary lesions: LEONARDO Study. Cardiovasc Revasc Med 2015;16:141-6.
98. Ojeda S, Azzalini L, Suárez de Lezo J, et al. Excimer laser coronary atherectomy for uncrossable coronary lesions. A multicenter registry. Catheter Cardiovasc Interv 2021;98:1241-9.
99. Kereiakes DJ, Hill JM, Ben-Yehuda O, Maehara A, Alexander B, Stone GW. Evaluation of safety and efficacy of coronary intravascular lithotripsy for treatment of severely calcified coronary stenoses: design and rationale for the disrupt CAD III trial. Am Heart J 2020;225:10-8.
100. Brinton TJ, Ali ZA, Hill JM, et al. Feasibility of shockwave coronary intravascular lithotripsy for the treatment of calcified coronary stenoses. Circulation 2019;139:834-6.
101. Yeoh J, Hill J. Intracoronary lithotripsy for the treatment of calcified plaque. Interv Cardiol Clin 2019;8:411-24.
102. Ali ZA, Brinton TJ, Hill JM, et al. Optical coherence tomography characterization of coronary lithoplasty for treatment of calcified lesions: first description. JACC Cardiovasc Imaging 2017;10:897-906.
103. Ali ZA, Nef H, Escaned J, et al. Safety and effectiveness of coronary intravascular lithotripsy for treatment of severely calcified coronary stenoses: the disrupt CAD II study. Circ Cardiovasc Interv 2019;12:e008434.
104. Saito S, Yamazaki S, Takahashi A, et al. Intravascular lithotripsy for vessel preparation in severely calcified coronary arteries prior to stent placement - primary outcomes from the Japanese disrupt CAD IV study. Circ J 2021;85:826-33.
105. Emori H, Shiono Y, Kuriyama N, et al. Calcium fracture after intravascular lithotripsy as assessed with optical coherence tomography. Circ J 2023;87:799-805.
106. Kawai K, Sato Y, Hakoma JY, et al. CRT-100.41 histology, OCT, and Micro-CT evaluation of coronary calcification in atherosclerotic cadaver arteries treated with intravascular lithotripsy. JACC Cardiovasc Interv 2023;16:S2.
107. Hofmann FJ, Dörr O, Blachutzik F, et al. First case report of fully robotically assisted lithotripsy in heavily calcified left main stenosis. Eur Heart J Case Rep 2023;7:ytac427.
108. Sheikh AS, Connolly DL, Abdul F, Varma C, Sharma V. Intravascular lithotripsy for severe coronary calcification: a systematic review. Minerva Cardiol Angiol 2023;71:643-52.
109. Alfonso F, Bastante T, Antuña P, et al. Coronary lithoplasty for the treatment of undilatable calcified de novo and in-stent restenosis lesions. JACC Cardiovasc Interv 2019;12:497-9.
110. Huisman J, van der Heijden LC, Kok MM, et al. Two-year outcome after treatment of severely calcified lesions with newer-generation drug-eluting stents in acute coronary syndromes: a patient-level pooled analysis from TWENTE and DUTCH PEERS. J Cardiol 2017;69:660-5.
111. Sugiyama T, Yamamoto E, Fracassi F, et al. Calcified plaques in patients with acute coronary syndromes. JACC Cardiovasc Interv 2019;12:531-40.
112. Torii S, Sato Y, Otsuka F, et al. Eruptive calcified nodules as a potential mechanism of acute coronary thrombosis and sudden death. J Am Coll Cardiol 2021;77:1599-611.
113. Alfonso F, Cuesta J, Bastante T, Aguilera MC, Benedicto A, Rivero F. In-Stent restenosis caused by a calcified nodule: a novel pattern of neoatherosclerosis. Can J Cardiol 2016;32:830.e1-3.
114. Mori H, Finn AV, Atkinson JB, Lutter C, Narula J, Virmani R. Calcified nodule: an early and late cause of in-stent failure. JACC Cardiovasc Interv 2016;9:e125-6.
115. Furuse E, Tanabe J, Tajiri M, Kawanaka H, Shimizu W. In-stent restenosis caused by calcified nodule 11 years after paclitaxel eluting stent implantation treated with drug-coated balloon following rotational atherectomy. Cardiovasc Interv Ther 2020;35:302-3.
116. Nagata T, Minami Y, Katsura A, et al. Optical coherence tomography factors for adverse events in patients with severe coronary calcification. Int J Cardiol 2023;376:28-34.
117. Pengchata P, Pongakasira R, Wongsawangkit N, Phichaphop A, Wongpraparut N. Characteristics and pattern of calcified nodule and/or nodular calcification detected by intravascular ultrasound on the device-oriented composite endpoint (DoCE) in patients with heavily calcified lesions who underwent rotational atherectomy-assisted percutaneous coronary intervention. J Interv Cardiol 2023;2023:6456695.
118. Allali A, Richardt G, Toelg R, et al. High-speed rotational atherectomy versus modified balloons for plaque preparation of severely calcified coronary lesions: two-year outcomes of the randomised PREPARE-CALC trial. EuroIntervention 2023;18:e1365-7.
119. Han FJ, Zheng HJ, Zheng XZ, et al. Efficacy of intravascular ultrasound-guided rotational atherectomy combined with cutting balloon for pretreatment of severe coronary artery calcified lesions. Nan Fang Yi Ke Da Xue Xue Bao 2021;41:1044-9.
120. McLaughlin TJ, Sachdeva R, Kumar G. First United States experience with rota-shock: a case series. Cardiovasc Revasc Med 2022;40S:209-13.
121. Buono A, Basavarajaiah S, Choudhury A, et al. “RotaTripsy” for severe calcified coronary artery lesions: insights from a real-world multicenter cohort. Cardiovasc Revasc Med 2022;37:78-81.
122. Dwivedi P, Dhulipala V, Kumar KR, et al. Efficacy and safety of an upfront RotaTripsy strategy in the treatment of de novo and in-stent restenosis cases. J Invasive Cardiol 2023;35:E70-4.
123. Wong B, Kam KKH, So CY, et al. Synergistic coronary artery calcium modification with combined atherectomy and intravascular lithotripsy. J Invasive Cardiol 2023;35:E128-35.
124. Jurado-Román A, García A, Moreno R. ELCA-tripsy: combination of laser and lithotripsy for severely calcified lesions. J Invasive Cardiol 2021;33:E754-5.
Cite This Article
Export citation file: BibTeX | RIS
OAE Style
Russo D, Massaro G, Benedetto D, Russo G, Canova P, Pescetelli I, Fiocca L, Romano M, Lettieri C, Chiricolo G, Sangiorgi GM. Contemporary approach to heavily calcified lesions: tools of the trade, challenges, and pitfalls. Vessel Plus 2024;8:9. http://dx.doi.org/10.20517/2574-1209.2023.75
AMA Style
Russo D, Massaro G, Benedetto D, Russo G, Canova P, Pescetelli I, Fiocca L, Romano M, Lettieri C, Chiricolo G, Sangiorgi GM. Contemporary approach to heavily calcified lesions: tools of the trade, challenges, and pitfalls. Vessel Plus. 2024; 8: 9. http://dx.doi.org/10.20517/2574-1209.2023.75
Chicago/Turabian Style
Russo, Debora, Gianluca Massaro, Daniela Benedetto, Giulio Russo, Paolo Canova, Irene Pescetelli, Luigi Fiocca, Michele Romano, Corrado Lettieri, Gaetano Chiricolo, Giuseppe Massimo Sangiorgi. 2024. "Contemporary approach to heavily calcified lesions: tools of the trade, challenges, and pitfalls" Vessel Plus. 8: 9. http://dx.doi.org/10.20517/2574-1209.2023.75
ACS Style
Russo, D.; Massaro G.; Benedetto D.; Russo G.; Canova P.; Pescetelli I.; Fiocca L.; Romano M.; Lettieri C.; Chiricolo G.; Sangiorgi GM. Contemporary approach to heavily calcified lesions: tools of the trade, challenges, and pitfalls. Vessel Plus. 2024, 8, 9. http://dx.doi.org/10.20517/2574-1209.2023.75
About This Article
Special Issue
Copyright
Data & Comments
Data
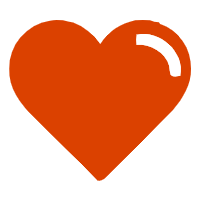

Comments
Comments must be written in English. Spam, offensive content, impersonation, and private information will not be permitted. If any comment is reported and identified as inappropriate content by OAE staff, the comment will be removed without notice. If you have any queries or need any help, please contact us at support@oaepublish.com.