Thermal ablation of metastatic disease to the musculoskeletal system
Abstract
A variety of indications have been published regarding the use of percutaneous thermal ablation for treating tumors of the musculoskeletal system, including bone and soft tissue lesions, benign and malignant lesions, and primary and metastatic tumors. In the appropriately selected patient, the advantages of percutaneous thermal ablation include decreased morbidity, decreased cost, and shorter hospitalization stays compared to surgery. The number of different thermal ablation modalities is increasing, and each modality has its advantages and disadvantages. Studies directly comparing the effectiveness of the various thermal ablation modalities are sparse, however, so the choice of ablation modality often depends on availability, user preference, and local expertise. Although the list of uses for percutaneous thermal ablation is ever-expanding, in this article, we will discuss the two most well-established indications, which are palliation of pain attributed to bone and soft tissue metastases and local control of oligometastatic disease. Numerous clinical trials have shown percutaneous thermal ablation to be an effective method of palliating pain due to bone and soft tissue metastases and of achieving local control in the setting of oligometastatic disease with low rates of complication.
Keywords
INTRODUCTION
Percutaneous thermal ablation is a minimally invasive treatment in which one or more specialized needles - ablation probes - are inserted into a target lesion(s) using image guidance. These ablation probes are attached to a device that delivers lethal thermal energy to a zone around the tip of the ablation probe known as the ablation zone. The size and shape of the ablation zone are determined by the size of the active tip of the ablation probe and the type of thermal energy being delivered, such as radiofrequency ablation versus cryoablation. Multiple ablation probes can often be used simultaneously to craft customized ablation zones that conform to the size and shape of the lesion being treated. In the appropriately selected patient, advantages of percutaneous thermal ablation include decreased morbidity, decreased cost, and shorter hospitalization stays compared to surgery[1].
A variety of indications have been published regarding the use of percutaneous thermal ablation for treating tumors of the musculoskeletal system. In this review, we will focus on the two most well-established indications: palliation of painful bone and soft tissue metastases, and local control of oligometastatic disease. Other published indications include treatment of benign tumors (specifically, osteoid osteoma[1,2], chondroblastoma[3,4], osteoblastoma[5-7], extra-abdominal desmoid tumor[8-13], and giant cell tumor[14,15]) and, more recently, treatment of abdominal wall endometriosis[13,16,17].
INDICATIONS FOR THERMAL ABLATION
Palliation of painful bone and soft tissue metastases
In patients with breast, prostate, and lung cancer, up to 85% have musculoskeletal metastases at the time of death[18], and approximately 50%-80% have painful metastases[19,20]. Radiation therapy is the standard treatment for these symptomatic lesions; however, the pain can be resistant to or recur after treatment, with only approximately one-third of patients able to experience long-lasting pain relief[21,22]. Analgesics (opioid and non-opioid), osteoclast inhibitors (e.g., bisphosphonates, denosumab), and systemic anticancer therapy (chemotherapy, targeted therapies, hormone therapy) also play important roles in the treatment of painful musculoskeletal metastases. Surgery is typically reserved for lesions associated with pathologic fracture, mechanical spinal instability, or epidural spinal cord compression[23].
Over the past two decades, clinical trials have shown percutaneous thermal ablation to be an effective, safe, and durable method of pain palliation for bone and soft tissue metastases. This treatment has emerged as an important therapeutic option for patients with persistent or recurrent localized pain from bone and soft tissue metastases who are not candidates for surgery or reirradiation. Determining whether thermal ablation is appropriate, however, requires a multidisciplinary team approach involving medical oncology, radiation oncology, orthopedic oncology, and interventional radiology. Patients selected for ablation should have at least moderate pain (defined as 4 or more on a standardized 10-point pain scale) related to a limited number of metastases seen on cross-sectional imaging that correlate to the site(s) of pain on physical examination[24]. For patients with at most mild pain (0-3 on a standardized 10-point pain scale), analgesics may be more beneficial than ablation.
Lesions best suited for percutaneous thermal ablation include those that are osteolytic, mixed osteolytic/osteoblastic, or those that have a prominent soft tissue component. Purely osteoblastic lesions may also be targeted, but they can be technically challenging to access in the presence of intact bone. The junction between the metastasis and the bone (the “bone-tumor interface”) is felt to be the primary source of pain, so in order to palliate pain effectively, ablation probes should be positioned with a primary focus towards ablating the bone-tumor interface and not necessarily the center or even the entirety of the lesion
Figure 1. 63-year-old female with stage IV renal cell carcinoma. (A) Axial CT image through the pelvis shows a large destructive lytic metastasis involving the posterior aspect of the left hip resulting in severe pain (circle). (B) Axial CT image during cryoablation of the left hip metastasis for pain palliation shows one of multiple cryoablation probes inserted into the lesion using a posterior approach (arrow). The ovoid low attenuation ablation zone is visible (arrowheads) and covers the bone-tumor interface.
Figure 2. 49-year-old female with stage IV breast cancer. (A) Coronal maximum intensity projection image from FDG PET study shows extensive FDG-avid osseous metastases throughout the spine, pelvis, and ribs. FDG activity in the renal collecting systems, urinary bladder, gastrointestinal tract, and brain is normal. (B) Axial fused FDG PET-CT image of the chest shows an FDG-avid metastasis in the sternum resulting in severe pain (circle). There is a subtle pathologic fracture obscured by the FDG activity. The lesion lies less than 1 cm away from the skin surface. (C) Axial CT image during radiofrequency ablation of the sternal metastasis for pain palliation shows the radiofrequency probe within the lesion (dotted arrow). Because of close proximity of the lesion and the planned radiofrequency ablation zone to the skin surface, a spinal needle was inserted into the subcutaneous tissues anterior to the sternum (solid arrow), and hydrodisplacement was performed to displace the skin away from the sternal metastasis and the ablation zone (*). (D) Follow-up axial fused FDG PET-CT image following treatment shows marked decrease in FDG activity (circle), with residual FDG activity due to the healing pathologic fracture. The patient experienced a moderate reduction in her presenting pain.
Successful pain palliation following percutaneous thermal ablation of bone and soft tissue metastases has been well demonstrated across multiple studies[27-42]. A 2019 systematic review of 11 papers (3 on radiofrequency, 2 on cryoablation, 1 on microwave, and 5 on focused ultrasound) showed mean pain reduction ranging from 26% to 91% at 4 weeks and 16% to 95% at 12 weeks, with all techniques achieving pain relief after 1 and 3 months in 91% and 95% of patients, respectively[41]. More recently, the MOTION trial (Multicenter Study of Cryoablation for Palliation of Painful Bone Metastases), which included 66 patients, showed mean pain reduction by 2 points (on a 10-point scale) by week 1 and 2.61 points by week 8, with improved quality of life, stabilized opioid doses, and maintained functional status over 6 months[30]. The OPuS One Study, which included 100 patients treated for bone metastases using radiofrequency ablation, showed rapid (within 3 days) and statistically significant pain improvement, with sustained long-term relief through 6 months[40].
There are no randomized controlled trials comparing the different thermal ablation approaches for the management of symptomatic bone and soft tissue metastases. Some studies have suggested cryoablation to be superior to radiofrequency ablation in achieving decreased opioid requirements, shorter hospital stays, and complete pain response[43,44]. The choice of ablation modality, however, depends on multiple factors, including availability, user preference, and local expertise.
Local tumor control (oligometastatic disease)
Oligometastatic disease (5 or fewer metastases) is a state in which patients may still be cured with focal therapy, which, although aggressive, avoids or postpones the potential side effects of systemic therapy, including chemotherapy, hormonal therapy, immunotherapy, or other targeted pharmacologic therapies. In patients with limited bone and soft tissue metastases, percutaneous thermal ablation may be used to achieve local tumor control or even disease remission[23,45,46]. Surgical resection, though used to treat limited metastases in other locations such as liver, lung, and kidney, is much less common for musculoskeletal metastases due to the morbidity of resection and the potential for functional loss[45]. Stereotactic body radiotherapy (SBRT) has been increasingly used to treat oligometastatic bone disease[47].
As with palliation of painful metastatic disease, percutaneous thermal ablation may be used in select patients with oligometastatic disease [Figure 3]. The criteria for choosing patients for ablation of oligometastatic disease are not well established and, like palliation of painful bone and soft tissue metastatic disease, should involve a multidisciplinary team approach. Patients who are not candidates for radiation therapy, patients with lesions unresponsive to radiation therapy, or patients with lesions that have developed in previously irradiated fields may be good candidates for percutaneous thermal ablation[45]. Unlike ablation for palliation of painful bone and soft tissue metastatic disease, which targets the bone-tumor interface, ablating to achieve durable local tumor control with minimal risk of residual or recurrent disease requires creating an ablation zone that encompasses the entire lesion plus a margin [Figure 4][1]. Therefore, the target lesions should be located 10 mm or more from critical structures[46]. Additionally, lesions with large intratumoral vessels should be avoided, as the vessels can act as a thermal sink and dissipate the heat or cold away from the ablation zone, thus limiting the effectiveness of the ablation[19].
Figure 3. 47-year-old female with sacral chordoma. (A) Sagittal T1-weighted, (B) sagittal short tau inversion recovery (STIR), and (C) axial T2-weighted images of the lumbar spine show a marrow-replacing lesion in the L2 vertebral body, detected during routine imaging surveillance of the entire spine, consistent with metastatic disease (*). No other lesions were detected. Biopsy of the lesion revealed metastatic chordoma. The patient was asymptomatic from this lesion and presented for radiofrequency ablation of oligometastatic disease. (D) Prone axial CT image during radiofrequency ablation of the L2 lesion shows 2 radiofrequency probes inserted into the lesion using a bipedicular approach (arrows). Because of the midline location of the lesion within the vertebral body, a bipedicular approach was needed to ensure maximum ablative coverage of the lesion.
Figure 4. 36-year-old female with stage IV leiomyosarcoma. Axial (A) T1-weighted, (B) T2-weighted fat-suppressed, and
Case series, most using cryoablation, have shown variable rates of tumor control for bone and soft tissue oligometastatic disease, ranging from 36% to 97%[46,48,49]. One series of 46 patients with 49 bone metastases (37 treated with cryoablation, 12 treated with radiofrequency) showed a local progression-free survival of 76.8% at 1 year and 71.7% at 2 years, irrespective of tumor histology, with bone metastases greater than 2 cm in size showing higher rates of local tumor progression[48]. In another single institution study examining musculoskeletal oligometastatic disease treated with cryoablation, local control was achieved in 45 of 52 tumors (87%) at a median follow-up of 21 months[49]. Just as with palliation of painful bone and soft tissue metastases, there are no randomized trials comparing the efficacy of the different thermal ablation modalities with respect to local tumor control for the management of oligometastatic disease.
MODALITIES OF THERMAL ABLATION
There are several ablation modalities in clinical use, but the two principal ablation modalities to date for bone and soft tissue metastases are radiofrequency ablation and cryoablation. For all image-guided ablation modalities, the patient is brought to the procedure room and positioned on the imaging table in a way that permits a safe trajectory from the skin surface to the lesion(s). CT is the most commonly used method of image guidance, but ultrasound and even MRI can be utilized under the appropriate circumstances. Procedural sedation or anesthesia is administered by the appropriate personnel with the goal of providing maximum comfort with no patient motion during a procedure which can last up to several hours for large or multiple lesions. After preliminary images are acquired to localize the tumor and identify a site and trajectory for probe placement, ablation probes are advanced sterilely into the target under image guidance. Ablation probes can be inserted directly into the lesion if it is composed of soft tissue, but they cannot be inserted directly into bone since they do not have cutting or drilling surfaces. In this situation, the lesion must first be accessed using separate cannulas or drills, after which the ablation probes can be inserted coaxially through the auxiliary instruments into the lesion. The generator to which the ablation probes are attached is then activated to allow the ablation probes to achieve target ablation temperatures and maintain them for a specified duration according to published ablation protocols, which vary according to ablation modality, equipment manufacturer, and target tissue type. Once tumor ablation is complete, the ablation probes and any accompanying instruments are removed. When hemostasis is achieved and wounds are dressed, the patient is transferred to the recovery room before being discharged back to the wards if the ablation is performed on an inpatient basis or to home or other facilities if the ablation is performed on an outpatient basis. Individual ablation modalities are discussed in greater detail in the following sections.
Radiofrequency ablation
Radiofrequency ablation (RFA) uses high-frequency alternating electric current to generate ionic agitation and frictional heating within target tissue, resulting in denaturing of proteins, damage to cell membranes, and ultimately cell death. There are monopolar and bipolar RFA systems. In monopolar RFA systems, each ablation probe contains a single electrode, which produces an elliptical zone of heating; thermal conduction expands the ablation zone into surrounding tissue, with temperature dropping precipitously as the distance from the probe tip increases[19]. Monopolar RFA systems require the use of grounding pads to complete the circuit through the body. In bipolar RFA systems, energy flows between two electrodes, which eliminates the need for grounding pads and creates a more focused ablation area[42]. Cell death occurs instantly at temperatures above 60 °C and within minutes at temperatures between 50 °C and 60 °C. Because of the insulating effects of cortical bone, RFA is less favored for blastic metastases[50]. Ablation zones cannot be visualized by CT or ultrasound but may be estimated using temperature-sensitive MR pulse sequences; however, it is impractical to perform all RFA procedures using MRI guidance[51]. Some RFA systems can accommodate multiple ablation probes, which allows the creation of larger ablation zones with some limited ability to customize their shape.
RFA may also be combined with cementoplasty to stabilize axial load-bearing bones (such as spine and acetabulum) and decrease the risk of pathologic fracture. Cementoplasty, which involves percutaneous instillation of polymethylmethacrylate cement into bone, may be performed immediately after heat-based ablation[46]. Studies have shown that cementoplasty of skeletal metastases, performed alone or in combination with percutaneous thermal ablation, results in pain palliation[1].
Potential risks of RFA include postablation syndrome (malaise and fatigue caused by the release of cytokines from tumoral cell death), a paradoxical increase in pain in the first week following the procedure, and subsequent fracture[19]. The risk of thermal injury to adjacent structures, including skin burns[1], may be minimized by careful probe positioning and insulation techniques.
Cryoablation
Cryoablation uses pressurized room-temperature argon gas delivered through an insulated probe. Rapid expansion of gas at the non-insulated probe tip causes rapid cooling, with temperatures reaching -100 °C within a few seconds. This results in the formation of a low-attenuation ice ball, which can be easily visualized on noncontrast CT or MRI. The ice ball contains both intracellular and extracellular ice. Intracellular ice causes mechanical shear injury to the cells. Extracellular ice dehydrates the cells and damages the cell membrane and cellular enzymes. These mechanisms occur simultaneously and result in cell death. The size of the ice ball depends on the length of the probe tip, the diameter of the cryoprobe, and the duration of freezing. Most cryoablation systems allow the simultaneous use of multiple ablation probes, placed 1.5-2 cm apart, to provide the best geometric coverage of the tumor. For bone and soft tissue metastases, a standard freeze-thaw-freeze cycle of 10-5-10 min is performed for each lesion, with intermittent imaging obtained typically every 2-3 min to monitor ice ball formation and ensure safe distancing from any nearby critical structures. Complete cell death is achieved at temperatures below
There are several advantages of cryoablation over RFA. Firstly, the ablation zone is visible on cross-sectional imaging and therefore can be monitored in real time during the treatment. This allows for greater confidence and safety in treating tumor margins, especially when operating near critical structures. Secondly, multiple cryoprobes, typically more than 2, may be placed simultaneously, which permits the creation of larger ablation zones with more customizable shapes. Thirdly, the ice ball penetrates deeply into bone, allowing effective treatment of osteoblastic metastases, whereas RF energy penetrates poorly into sclerotic bone. Lastly, cryoablation patients experience less pain during the immediate posttreatment period compared to RFA[43,44], possibly because the freezing temperatures achieved during treatment may provide a degree of anesthesia (cryoanalgesia)[50]. Disadvantages of cryoablation include greater procedural time and increased cost compared to RFA[51]. The lack of an insulating effect of cortical bone on cryoablation can lead to a deeper propagation of ice through the bone, which can be a disadvantage when ablating near the spinal canal.
Cryoablation of bone and soft tissue metastases has been shown to be very safe, with low rates of complications[52,53]. A large retrospective case series including 239 patients and 320 bone tumors showed a major complication rate of 2.5%, with pathologic fracture being the most common complication (1.2%). Other major complications included tumor seeding, arterial bleeding, ablation site infection, and severe hypotension, each occurring in one of 320 procedures (0.3%). Risk factors for major complications included: the use of 3 cryoprobes, patient age > 70 years, and cryoablation of long bones. Minor complications included post-procedural pain, temporary paresthesia, and peripheral neuropathy. The total complication rate was 9.1%[52]. Postablation syndrome, described above in the section on RFA, can also occur after cryoablation.
Cryoablation may also be combined with cementoplasty to decrease the risk of pathologic fracture. To improve filling of the tumor cavity, cementoplasty is most often done one day or more after cryoablation to allow for adequate tissue thawing and necrosis[54].
Microwave ablation
Microwave ablation (MWA) relies on electromagnetic energy transmitted through antennae in a microwave probe to force water molecules to oscillate, generating heat. Unlike RF energy, microwaves can propagate through materials with low or zero conductivity, resulting in larger and more uniform ablation zones. This property also makes MWA relatively immune to heat sink effects and well-suited for treating tumors with high water content (e.g., large vascular tumors). MWA can achieve cell death temperatures faster than other ablation techniques[50].
A systematic review evaluating MWA protocol, safety, and efficacy in treating bone tumors, which included seven non-comparative studies and 269 malignant bone tumors, showed that MWA was effective at achieving pain relief at 4-6 months[55]. The authors concluded that although MWA appeared safe, further studies are needed, as there is large heterogeneity in ablation protocols, and more evidence is needed.
Microwave ablation may also be combined with cementoplasty to alleviate pain and reduce the risk of pathologic fracture[56].
Focused ultrasound and laser ablation
Focused ultrasound is a relatively newer and completely non-invasive thermal ablation technique in which high-intensity ultrasound waves are focused on a small target area (usually under MR thermometry guidance), resulting in tissue heating and cell death. Focused ultrasound has been shown to be effective in palliating painful bone metastases[39,57,58].
Laser ablation is another heat-based thermal ablation technique in which infrared energy delivered through laser fibers causes precisely targeted tissue coagulation/cell death. The use of laser ablation in the musculoskeletal system has largely been limited to the treatment of benign lesions (e.g., osteoid osteoma, vascular malformations)[50].
Intraoperative ablation
Thermal ablation may also be performed intraoperatively as an adjunct/adjuvant treatment technique to improve surgical margins. Intraoperative thermal ablation has already been described in the treatment of non-musculoskeletal tumors (e.g., liver[59], lung[60], and brain[61]). The underlying principles may also be applied in the treatment of musculoskeletal tumors.
DISCUSSION
Over the past two decades, percutaneous thermal ablation has emerged as an effective and promising tool for treating metastatic disease in bone and soft tissue. It is typically performed on an outpatient basis with relatively short recovery times and low complication rates and can be performed either alone or in conjunction with surgery, radiation, or systemic therapy. Since there are few guidelines prescribing which lesions should be treated with thermal ablation, deciding which patients will benefit from this treatment requires thoughtful multidisciplinary discussion amongst the patient’s medical oncologist, radiation oncologist, orthopedic oncologist, and interventional radiologist. The two most studied and well-established indications are palliation of painful metastases and local control/cure of oligometastatic disease. The choice of ablation modality depends on lesion character, size, and location, as well as user preference, experience, and equipment availability. There are no randomized controlled trials showing one ablation modality to be superior to another. Thus, the decision on which modality to use lies with the interventionalist, and the advantages and disadvantages of each should be considered.
It is the authors’ experience that the main factor influencing the choice of ablation modality is lesion size. Larger lesions are better suited to cryoablation, since individual cryoablation probes can generate ablation zones up to 6 cm × 4 cm × 4 cm, and some cryoablation systems can accommodate up to 20 probes simultaneously, allowing the possibility of creating very large ablation zones. By contrast, the largest ablation zones generated by RFA probes are on the order of 3 cm × 2 cm × 2 cm, and since RFA systems typically accommodate only up to two probes simultaneously, using an RFA system to create an ablation zone of comparable size to a multi-cryoablation probe ablation zone would require repositioning the RFA probes within the target multiple times to perform multiple serial ablations, which could be prohibitively time-consuming. On the other hand, the minimum ablation zone size of an RFA system is approximately
Summary of the main differences between radiofrequency ablation and cryoablation
Radiofrequency ablation | Cryoablation | |
Ablation zone visible? | No | Yes for soft tissue, not typically for bone |
Lesion size | Good for lesions < 3 cm, not well-suited for lesions | Good for lesions > 5 cm, not well-suited for lesions < 1 cm |
Lytic vs. blastic lesions | Not as effective for blastic lesions | No difference between lytic and blastic lesions |
Spine lesions | Intact cortex can protect against damage to adjacent structures, e.g. nerve and spinal cord | Intact cortex is not protective against damage to adjacent structures |
Combine with cementoplasty? | Can be performed at the same time | Best if delayed by 1-2 days |
Ablation time | < 15 min | At least 25 min for standard musculoskeletal ablation protocol |
Procedure cost | Generally shorter procedure room time, fewer probes needed, each less expensive | Generally longer procedure room time, more probes needed for large lesions, each more expensive |
Post-procedure pain | Variable, usually mild | Variable, usually less than RFA due to cryoanalgesia |
Clinical trials have shown thermal ablation to be effective in achieving palliation of painful metastases; results have been more variable when trying to achieve local control for oligometastatic disease. In most series, the proportion of subjects being treated with sarcoma metastases is very small, so it is unknown whether this variability can be attributed to differences in tissue type. However, since the cell death mechanisms induced by thermal ablation do not act upon the DNA machinery of the cell, it seems unlikely that the resistance mechanisms that occur with chemotherapy and radiation therapy and certain tissue types will apply to thermal ablation.
CONCLUSION
The use of percutaneous thermal ablation is ever expanding, and thermal ablation should be considered and offered to patients when discussing treatment options, particularly with respect to palliation of painful bone and soft tissue metastases and local control of oligometastatic disease. Because of the dearth of randomized controlled studies, there are many unanswered and incompletely answered questions that are worthy of pursuit and additional study. As more clinicians incorporate this tool into their arsenal, more data could lead to new indications and more established guidelines for effective use.
DECLARATIONS
Authors’ contributions
Literature search, manuscript writing: Xia E
Conceptualization, manuscript writing, manuscript review and editing, figures and tables: Huang AJ
Availability of data and materials
Not applicable.
Financial support and sponsorship
None.
Conflicts of interest
Both authors declared that there are no conflicts of interest.
Ethical approval and consent to participate
Not applicable.
Consent for publication
Not applicable.
Copyright
© The Author(s) 2023.
REFERENCES
1. Rosenthal D, Callstrom MR. Critical review and state of the art in interventional oncology: benign and metastatic disease involving bone. Radiology 2012;262:765-80.
2. Rosenthal DI, Alexander A, Rosenberg AE, Springfield D. Ablation of osteoid osteomas with a percutaneously placed electrode: a new procedure. Radiology 1992;183:29-33.
3. Erickson JK, Rosenthal DI, Zaleske DJ, Gebhardt MC, Cates JM. Primary treatment of chondroblastoma with percutaneous radio-frequency heat ablation: report of three cases. Radiology 2001;221:463-8.
4. Rybak LD, Rosenthal DI, Wittig JC. Chondroblastoma: radiofrequency ablation-alternative to surgical resection in selected cases. Radiology 2009;251:599-604.
5. Cazzato RL, Auloge P, Dalili D, et al. Percutaneous image-guided cryoablation of osteoblastoma. Am J Roentgenol 2019;213:1157-62.
6. Beyer T, van Rijswijk CSP, Villagrán JM, et al. European multicentre study on technical success and long-term clinical outcome of radiofrequency ablation for the treatment of spinal osteoid osteomas and osteoblastomas. Neuroradiology 2019;61:935-42.
7. Weber MA, Sprengel SD, Omlor GW, et al. Clinical long-term outcome, technical success, and cost analysis of radiofrequency ablation for the treatment of osteoblastomas and spinal osteoid osteomas in comparison to open surgical resection. Skeletal Radiol 2015;44:981-93.
8. Ilaslan H, Schils J, Joyce M, Marks K, Sundaram M. Radiofrequency ablation: another treatment option for local control of desmoid tumors. Skeletal Radiol 2010;39:169-73.
9. Ghanouni P, Dobrotwir A, Bazzocchi A, et al. Magnetic resonance-guided focused ultrasound treatment of extra-abdominal desmoid tumors: a retrospective multicenter study. Eur Radiol 2017;27:732-40.
10. Schmitz JJ, Schmit GD, Atwell TD, et al. Percutaneous cryoablation of extraabdominal desmoid tumors: a 10-year experience. Am J Roentgenol 2016;207:190-5.
11. Tremblay KR, Lea WB, Neilson JC, King DM, Tutton SM. Percutaneous cryoablation for the treatment of extra-abdominal desmoid tumors. J Surg Oncol 2019;120:366-75.
12. Kujak JL, Liu PT, Johnson GB, Callstrom MR. Early experience with percutaneous cryoablation of extra-abdominal desmoid tumors. Skeletal Radiol 2010;39:175-82.
13. Parvinian A, Welch BT, Callstrom MR, Kurup AN. Trends in musculoskeletal ablation: emerging indications and techniques. Tech Vasc Interv Radiol 2020;23:100678.
14. Pimolsanti R, Wongkajornsilpa A, Chotiyarnwong P, Asavamongkolku A, Waikakul S. Effects of thermoablation with or without caffeine on giant cell tumour of bone. J Orthop Surg 2015;23:95-9.
15. Luna LP, Sankaran N, Ehresman J, Sciubba DM, Khan M. Successful percutaneous treatment of bone tumors using microwave ablation in combination with Zoledronic acid infused PMMA cementoplasty. J Clin Neurosci 2020;76:219-25.
16. Maillot J, Brun JL, Dubuisson V, Bazot M, Grenier N, Cornelis FH. Mid-term outcomes after percutaneous cryoablation of symptomatic abdominal wall endometriosis: comparison with surgery alone in a single institution. Eur Radiol 2017;27:4298-306.
17. Dibble EH, D'Amico KC, Bandera CA, Littrup PJ. Cryoablation of abdominal wall endometriosis: a minimally invasive treatment. Am J Roentgenol 2017;209:690-6.
18. Nielsen OS, Munro AJ, Tannock IF. Bone metastases: pathophysiology and management policy. J Clin Oncol 1991;9:509-24.
19. Rybak LD. Fire and ice: thermal ablation of musculoskeletal tumors. Radiol Clin North Am 2009;47:455-69.
20. Simon CJ, Dupuy DE. Percutaneous minimally invasive therapies in the treatment of bone tumors: thermal ablation. Semin Musculoskelet Radiol 2006;10:137-44.
21. Chow E, Zeng L, Salvo N, Dennis K, Tsao M, Lutz S. Update on the systematic review of palliative radiotherapy trials for bone metastases. Clin Oncol 2012;24:112-24.
22. Sze WM, Shelley M, Held I, Mason M. Palliation of metastatic bone pain: single fraction versus multifraction radiotherapy - a systematic review of the randomised trials. Cochrane Database Syst Rev 2004;2002:CD004721.
23. Kurup AN, Callstrom MR. Ablation of musculoskeletal metastases: pain palliation, fracture risk reduction, and oligometastatic disease. Tech Vasc Interv Radiol 2013;16:253-61.
24. Kurup AN, Callstrom MR. Ablation of skeletal metastases: current status. J Vasc Interv Radiol 2010;21:S242-50.
25. Tsoumakidou G, Buy X, Garnon J, Enescu J, Gangi A. Percutaneous thermal ablation: how to protect the surrounding organs. Tech Vasc Interv Radiol 2011;14:170-6.
26. Kurup AN, Schmit GD, Morris JM, et al. Avoiding complications in bone and soft tissue ablation. Cardiovasc Intervent Radiol 2017;40:166-76.
27. Goetz MP, Callstrom MR, Charboneau JW, et al. Percutaneous image-guided radiofrequency ablation of painful metastases involving bone: a multicenter study. J Clin Oncol 2004;22:300-6.
28. Dupuy DE, Liu D, Hartfeil D, et al. Percutaneous radiofrequency ablation of painful osseous metastases: a multicenter American college of radiology imaging network trial. Cancer 2010;116:989-97.
29. Wallace AN, Greenwood TJ, Jennings JW. Radiofrequency ablation and vertebral augmentation for palliation of painful spinal metastases. J Neurooncol 2015;124:111-8.
30. Jennings JW, Prologo JD, Garnon J, et al. Cryoablation for palliation of painful bone metastases: the MOTION multicenter study. Radiol Imaging Cancer 2021;3:e200101.
31. Callstrom MR, Dupuy DE, Solomon SB, et al. Percutaneous image-guided cryoablation of painful metastases involving bone: multicenter trial. Cancer 2013;119:1033-41.
32. Prologo JD, Passalacqua M, Patel I, Bohnert N, Corn DJ. Image-guided cryoablation for the treatment of painful musculoskeletal metastatic disease: a single-center experience. Skeletal Radiol 2014;43:1551-9.
33. Tomasian A, Wallace A, Northrup B, Hillen TJ, Jennings JW. Spine cryoablation: pain palliation and local tumor control for vertebral metastases. AJNR Am J Neuroradiol 2016;37:189-95.
34. Kastler A, Alnassan H, Pereira PL, et al. Analgesic effects of microwave ablation of bone and soft tissue tumors under local anesthesia. Pain Med 2013;14:1873-81.
35. Pusceddu C, Sotgia B, Fele RM, Melis L. Treatment of bone metastases with microwave thermal ablation. J Vasc Interv Radiol 2013;24:229-33.
36. Khanmohammadi S, Noroozi A, Yekaninejad MS, Rezaei N. Cryoablation for the palliation of painful bone metastasis: a systematic review. Cardiovasc Intervent Radiol 2023;46:1469-82.
37. Bitton RR, Rosenberg J, LeBlang S, et al. MRI-guided focused ultrasound of osseous metastases: treatment parameters associated with successful pain reduction. Invest Radiol 2021;56:141-6.
38. Gianfelice D, Gupta C, Kucharczyk W, Bret P, Havill D, Clemons M. Palliative treatment of painful bone metastases with MR imaging-guided focused ultrasound. Radiology 2008;249:355-63.
39. Hurwitz MD, Ghanouni P, Kanaev SV, et al. Magnetic resonance-guided focused ultrasound for patients with painful bone metastases: phase III trial results. J Natl Cancer Inst 2014;106:dju082.
40. Levy J, Hopkins T, Morris J, et al. Radiofrequency ablation for the palliative treatment of bone metastases: outcomes from the multicenter OsteoCool tumor ablation post-market study (OPuS One Study) in 100 patients. J Vasc Interv Radiol 2020;31:1745-52.
41. Gennaro N, Sconfienza LM, Ambrogi F, Boveri S, Lanza E. Thermal ablation to relieve pain from metastatic bone disease: a systematic review. Skeletal Radiol 2019;48:1161-9.
42. Razakamanantsoa L, Kurup AN, Callstrom MR, Jennings JW, Cornelis FH. Bone ablations in peripheral skeleton: Rationale, techniques and evidence. Tech Vasc Interv Radiol 2022;25:100804.
43. Thacker PG, Callstrom MR, Curry TB, et al. Palliation of painful metastatic disease involving bone with imaging-guided treatment: comparison of patients' immediate response to radiofrequency ablation and cryoablation. Am J Roentgenol 2011;197:510-5.
44. Zugaro L, DI Staso M, Gravina GL, et al. Treatment of osteolytic solitary painful osseous metastases with radiofrequency ablation or cryoablation: a retrospective study by propensity analysis. Oncol Lett 2016;11:1948-54.
45. Kurup AN, Callstrom MR. Expanding role of percutaneous ablative and consolidative treatments for musculoskeletal tumours. Clin Radiol 2017;72:645-56.
46. Kurup AN, Morris JM, Callstrom MR. Ablation of musculoskeletal metastases. Am J Roentgenol 2017;209:713-21.
47. Thomas MC, Chen YH, Fite E, et al. Patient and treatment factors associated with improved local control and survival in oligometastatic bone disease: results from a large single-institution experience using stereotactic body radiation therapy. Int J Radiat Oncol Biol Phys 2022;114:747-61.
48. Luigi Cazzato R, Auloge P, De Marini P, et al. Percutaneous image-guided ablation of bone metastases: local tumor control in oligometastatic patients. Int J Hyperthermia 2018;35:493-9.
49. McMenomy BP, Kurup AN, Johnson GB, et al. Percutaneous cryoablation of musculoskeletal oligometastatic disease for complete remission. J Vasc Interv Radiol 2013;24:207-13.
50. Dalili D, Isaac A, Bazzocchi A, et al. Interventional techniques for bone and musculoskeletal soft tissue tumors: current practices and future directions - part I. ablation. Semin Musculoskelet Radiol 2020;24:692-709.
51. Callstrom MR, Kurup AN. Percutaneous ablation for bone and soft tissue metastases-why cryoablation? Skeletal Radiol 2009;38:835-9.
52. Auloge P, Cazzato RL, Rousseau C, et al. Complications of percutaneous bone tumor cryoablation: a 10-year experience. Radiology 2019;291:521-8.
54. Kurup AN, Schmit GD, Atwell TD, et al. Palliative percutaneous cryoablation and cementoplasty of acetabular metastases: factors affecting pain control and fracture risk. Cardiovasc Intervent Radiol 2018;41:1735-42.
55. Cazzato RL, de Rubeis G, de Marini P, et al. Percutaneous microwave ablation of bone tumors: a systematic review. Eur Radiol 2021;31:3530-41.
56. Zuo D, Sun M, Mu H, et al. O-arm-guided percutaneous microwave ablation and cementoplasty for the treatment of pelvic acetabulum bone metastasis. Front Surg 2022;9:929044.
57. Napoli A, De Maio A, Alfieri G, et al. Focused ultrasound and external beam radiation therapy for painful bone metastases: a phase II clinical trial. Radiology 2023;307:e211857.
58. Scipione R, Anzidei M, Bazzocchi A, Gagliardo C, Catalano C, Napoli A. HIFU for bone metastases and other musculoskeletal applications. Semin Intervent Radiol 2018;35:261-7.
59. Krul MF, Gerritsen SL, Vissers FL, et al. Radiofrequency versus microwave ablation for intraoperative treatment of colorectal liver metastases. Eur J Surg Oncol 2022;48:834-40.
60. Wolf FJ, Aswad B, Ng T, Dupuy DE. Intraoperative microwave ablation of pulmonary malignancies with tumor permittivity feedback control: ablation and resection study in 10 consecutive patients. Radiology 2012;262:353-60.
Cite This Article
Export citation file: BibTeX | RIS
OAE Style
Xia E, Huang AJ. Thermal ablation of metastatic disease to the musculoskeletal system. J Cancer Metastasis Treat 2023;9:36. http://dx.doi.org/10.20517/2394-4722.2023.26
AMA Style
Xia E, Huang AJ. Thermal ablation of metastatic disease to the musculoskeletal system. Journal of Cancer Metastasis and Treatment. 2023; 9: 36. http://dx.doi.org/10.20517/2394-4722.2023.26
Chicago/Turabian Style
Xia, Erwin, Ambrose J. Huang. 2023. "Thermal ablation of metastatic disease to the musculoskeletal system" Journal of Cancer Metastasis and Treatment. 9: 36. http://dx.doi.org/10.20517/2394-4722.2023.26
ACS Style
Xia, E.; Huang AJ. Thermal ablation of metastatic disease to the musculoskeletal system. J. Cancer. Metastasis. Treat. 2023, 9, 36. http://dx.doi.org/10.20517/2394-4722.2023.26
About This Article
Copyright
Data & Comments
Data
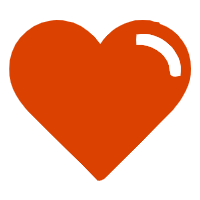

Comments
Comments must be written in English. Spam, offensive content, impersonation, and private information will not be permitted. If any comment is reported and identified as inappropriate content by OAE staff, the comment will be removed without notice. If you have any queries or need any help, please contact us at support@oaepublish.com.