A proteomic approach to better understand the role of human neutrophil peptides in the NSCLC microenvironment
Abstract
Aim: Although treatment of lung cancer, one of the deadliest diseases worldwide, with immune checkpoint inhibitors (ICIs) has shown promising outcomes, these survival outcomes are only observed in a relatively small subset of lung cancer patients. In a previous study, we elucidated that the presence of human neutrophil peptide (HNP) 1, 2 and 3 in non-small cell lung cancerous (NSCLC) biopsies is associated with a clinical response towards treatment with PD-1/PD-L1 immune checkpoint inhibitors. Furthermore, HNP1 has shown in vitro an immune-activated function towards lung cancer cells, but the specific role of HNP1 in (lung) cancer is still unknown. The aim of this study was to provide a better understanding of HNP1 in an NSCLC microenvironment.
Methods: To gain better insights into the role of HNP1 on cancer growth and to unravel immune responses, in vitro (SILAC-labelled) A549/PBMC (from three healthy donors) cocultures were set up and treated/not treated with HNP1. After 5 days, both secretome and cellular analysis using mass spectrometry were performed on these cocultures. After protein identification in all different tested conditions, pathway analyses (MetaCoreTM) were performed to investigate the biological significance of HNP1 stimulation on the A549/PBMC cocultures. The proteomic outcomes were confirmed by multiplex ELISA for a proinflammatory cytokine panel (TNF-α, IL-1β, IL-2, IL-4, IL-6, IL-12p70 and IL-18).
Results: A number of biological pathways and process networks were observed to be upregulated after treatment of the coculture with HNP1. HNP1 stimulation leads to an increase in pathways and proteins stimulating chemotaxis (including plasmin signaling, leucocyte recruitment, CCL2 and CXCL8 expression), proinflammatory cytokine secretion (including IL-1β, IL-6 and TNF-α), dendritic cell (DC) maturation, phagocytosis and antigen presentation, leading to a more efficient adaptive anti-tumoral immunity.
Conclusion: These results enhance our understanding of the role of HNP1 in the tumor microenvironment and suggest that HNP1 may be able to induce tumor necrosis by inducing prostimulatory immune responses.
Keywords
INTRODUCTION
Worldwide, lung cancer is still the most commonly diagnosed type of cancer. In 2020, more than 2.2 million patients were diagnosed with lung cancer. Moreover, lung cancer is responsible for the highest number of cancer-related deaths, accounting for 1.79 million deaths in 2020[1]. Recently, treatment with immune checkpoint inhibitors (ICIs) has been introduced as an additional treatment for lung cancer, with sustained clinical responses and significant survival advantages. Despite these clinical successes, a majority of the patients do not benefit from ICI treatment and are showing no or little response. To make matters worse, these nonresponding patients often suffer from serious side effects[2]. In PD-1/PD-L1 checkpoint inhibitor therapy, anticancer drugs that block the interaction of PD-1 and PD-L1 are administered to the patient. This prevents suppression of anti-tumoral T cell responses. Counterintuitively, it has been proven that patients with low to absent PD-L1 protein expression can still benefit from ICI therapy, questioning the value of PD-L1 as the sole biomarker to predict therapy outcomes[3,4]. In addition, the reliability of PD-L1 biomarker testing is hampered by the use of different monoclonal antibodies in clinical practice for PD-L1 detection, no defined threshold for PD-L1 expression and observed intratumor heterogeneity for PD-L1 expression[5]. Therefore, a lot of recent research has been focused on the identification and development of predictive biomarkers for ICI response in (lung) cancer patients, mostly with an emphasis on tumor mutational load, tumor-infiltrating lymphocytes and microsatellite instability. So far, however, a reliable, definitive predictive biomarker (set) is still lacking[5].
In a previous study, by using mass spectrometric approaches, we identified human neutrophil peptides (HNPs) 1, 2 and 3 as additional predictive biomarkers for a clinical response to anti-PD-(L)1 immunotherapy in non-small cell lung cancer (NSCLC) patients, with the NSCLC subtype accounting for the majority of lung cancer patients (85%)[6]. Furthermore, in the same study, we have reported in in vitro A549 cell cultures a decrease in tumor cell proliferation and an upregulated IFN-γ production upon HNP1 stimulation in the presence of peripheral blood mononuclear cells (PBMCs), while no decrease in tumor cell proliferation was observed in A549 monocultures after HNP1 stimulation, nor IFN-γ production in PBMC monocultures[6]. The question still remains: what caused the increased production of IFN-γ, as only little is known about the role of HNP(1) in cancer immunity[7].
While the three different HNPs are observed together in the analyzed pretreatment biopsies[6] and due to their very high sequence similarity (i.e., only differing in one amino acid), one reasonably can expect a very similar function(s)[7]. Therefore, in this study, we limited ourselves to HNP1 for functional analysis. HNP1-3 are α-defensins that, just like the β-defensins, have a cyclic structure characterized by three intramolecular disulfide bridges. The β-defensins are widely studied and are known to be important antimicrobial peptides with bacterial killing and growth inhibition capacities. They disrupt bacterial membranes, inhibit cell wall synthesis and neutralize secreted toxins[8]. α-defensins have been shown to exhibit similar antibacterial characteristics, but also antiviral, anticancer and even immune-modulatory activities have been described[7]. An overview of the described functions of the α-defensins is provided in Figure 1. Their activity seems to be dose-dependent: high HNP concentrations (micromolar range) may be responsible for the direct formation of pores in microbial (and some cancer cell) cell membranes, while low HNP concentrations (nanomolar) are thought to bind specific cell receptors having immune-modulating properties[7].
Figure 1. An overview of the already described functions of human neutrophil peptides 1, 2 and 3[7].
As mentioned earlier, the role of HNP(1) in cancer immunity is not fully elucidated. We have observed a reduced tumor cell proliferation and an increase in IFN-γ production in in vitro A549/PBMC cocultures. To study these complex interactions within the tumor microenvironment, secretome analyses are considered a valid approach for gaining a better understanding of cell communication, elucidating insights into cancer growth and progression and unraveling immune responses[9,10]. Also, proteomics to unravel the cellular content of the different cell types can provide crucial insights for a more comprehensive understanding of the underlying physiological significance within the tumor microenvironment[11]. In this study, we applied stable isotope labeling with amino acids in cell culture (SILAC)-based proteomic approaches on cocultures of PBMCs and tumor cells to trace the origin of the secreted and/or identified protein.
We analyzed the secretome from in vitro cell cultures of the A549 (SILAC-labeled) NSCLC cell line cocultured with PBMCs of three healthy donors, with and without HNP1 treatment. Afterwards, the secretome analysis was combined with whole-cell proteomics to provide deep proteomic profiling upon HNP1 stimulation of lung tumor cells cocultured with PBMCs. We observed different immune-related pathways that are clearly upregulated in two or all three donors after HNP1 stimulation of the in vitro cocultures. These interesting proteomic outcomes were confirmed by multiplex ELISA using a Meso Scale Discovery (MSD) proinflammatory cytokine panel (TNF-α, IL-1β, IL-2, IL-4, IL-6, IL-12p70 and IL-18). A schematic overview of the coculture analysis is presented in Figure 2. Combined with what is mentioned above, this study proves the power of proteomic approaches on cell (co)cultures to provide new molecular insights into the immune-activating role of HNP1 in a lung cancer immune-related context.
Figure 2. A schematic overview of the coculture analysis performed in this study. Created with BioRender.com.
MATERIALS AND METHODS
Cell cultures
The non-small-cell lung cancer cell line A549 (ATCC, Manassas, VA, USA) was cultured in stable isotope labeling with amino acids in cell culture (SILAC) medium comprising Dulbecco’s Modified Eagle Medium (DMEM, Thermo ScientificTM, Merelbeke, Belgium), supplemented with 10% non-heat inactivated fetal bovine serum (FBS) superior (Merck, Darmstadt, Germany), 1% penicillin/streptomycin (Thermo ScientificTM, Merelbeke, Belgium), 2 mM L-glutamine (Thermo ScientificTM, Merelbeke, Belgium) and the SILAC labeled amino acids 13C615N2 L-Lysine-2HCl, and 13C615N4 L-Arginine-HCl (Thermo ScientificTM, Merelbeke, Belgium), both added at a concentration of 0.1 g/L. SILAC-labeled A549 cells were maintained for at least five doubling times to achieve > 95% incorporation of the labeled amino acids, which was verified by proteome analysis. Cells were grown as monolayers and maintained in a humidified environment 5% CO2/95% air at 37 °C. Cell cultures were regularly tested for the absence of mycoplasma using the MYCOALERT® Mycoplasma detection kit (Lonza, Basel, Suisse).
Buffy coats derived from human whole blood donations, voluntarily provided by three healthy adult donors, were supplied by the Red Cross Flanders Blood Service (Mechelen, Belgium). Peripheral blood mononuclear cells (PBMCs) were isolated from the buffy coats by Ficoll-Paque Plus gradient (VWR, Leuven, Belgium). After isolation, rinsed PBMCs were resuspended in RPMI-1640 (Thermo ScientificTM, Merelbeke, Belgium), only supplemented with 2 mM L-glutamine.
Coculture set-up
To evaluate the effect of HNP1 on immune activation in a lung cancer context, A549 cell lines were seeded at 80,000 cells/well in 6 well plates (Corning, BD). After 24 h incubation of the A549, the SILAC-containing DMEM medium was aspirated and replaced with 800 µL serum-free DMEM, also referred to as conditioned cell medium (CCM), followed by setting up the coculture with PBMCs in a E/T ratio of 20:1, which seems to be perfectly suited for protein analysis, as proven earlier[6]. Then, the coculture was treated with HNP1
Secreted protein preparation
The conditioned medium was collected from the coculture(s) and centrifuged (150 g) for 5 min to remove cell debris, after which the supernatants were stored at -80 °C. Halt™ Protease and Phosphatase Inhibitor Cocktail (1:100) (Thermo ScientificTM, Merelbeke, Belgium) was added to inhibit any further enzyme activity. Proteins were precipitated with cold acetone (Thermo Fisher Acros Organics, Geel, Belgium) at
Suspension trapping (S-TrapTM) of secreted proteins
Protein pellets were resuspended in 5% sodium dodecyl sulfate (SDS, Sigma AldrichTM, Darmstadt, Germany), reduced for 1 h at 55 °C with tris(2-carboxyethyl) phosphine (TCEP, final concentration of
Cell pellet preparation
Cells were collected from the coculture(s) using a Versene [0.48 mM ethylenediamine tetraacetic acid
Suspension trapping (S-TrapTM) of cell lysates
Protein solutions were diluted to 1 µg/µL and further processed as described above with some adaptations of the volumes to the S-TrapTM micro-column. For loading the S-TrapTM micro-column, 165 µL S-Trap binding/washing buffer (90% aqueous methanol, 100 mM TEAB) was added to the sample and washes were performed with 150 µL S-Trap buffer. The column was centrifuged for 1 min at 4,000 g to trap proteins, digestion with trypsin was performed and tryptic peptides were eluted with twice 40 µL of 50% acetonitrile containing 0.1% formic acid[11]. Finally, peptides were vacuum dried.
LC-MS/MS analysis
The dried tryptic peptide fractions were dissolved in 20 µL mobile phase A (0.1% formic acid in HPLC-grade water (Biosolve, Valkenswaard, The Netherlands) at a concentration of 0.5 µg/µL. Each condition was measured in technical triplicates in a randomized order on the tims TOF Pro (Bruker Daltonik GmBH, Bremen, Germany) coupled to an Evosep one (Evosep). Peptides were separated using the 44 min default gradient with the column EV1106 (15 cm × 150 µm, 1.9 µm) on the Evosep One at a flow rate of
The column was online with a tims TOF Pro operating in positive ion mode, coupled with a CaptiveSpray ion source (Bruker Daltonik GmbH, Bremen, Germany). The tims TOF Pro was calibrated according to the manufacturer’s guidelines. The temperature of the ion transfer capillary was 180 °C. The Parallel Accumulation-Serial Fragmentation DDA method was used to select precursor ions for fragmentation with 1 TIMS-MS scan and 10 PASEF MS/MS scans, as described by Meier et al.[12]. The TIMS-MS survey scan was acquired between 0.70-1.45 V.s/cm2 and 100-1,700 m/z with a ramp time of 166 ms. The 10 PASEF scans contained on average 12 MS/MS scans per PASEF scan with a collision energy of 10 eV. Precursors with 1-6 charges were selected with the target value set to 20,000 a.u and intensity threshold to 2,500 a.u. Besides the standard PASEF method, all samples were also measured without a pre-defined polygon to include singly charged precursors. Precursors were dynamically excluded for 0.4 s. The tims TOF Pro was controlled by the OtofControl 5.1 software (Bruker Daltonik GmbH). Raw data were analyzed with the DataAnalysis 5.1 software (Bruker Daltonik).
Data analysis
Peptide identifications were generated using PEAKS Online X Software (Bioinformatics Solutions Inc., Waterloo, ON, Canada) with a parent mass error tolerance of 30 ppm and precursor mass error tolerance of 0.05 Da. The fixed modification was set at carbamidomethylation [at cysteine residue (+57.02 Da)] and possible variable post-translational modifications (PTMs) were set at a maximum of 3 variable PTMs per peptide and included oxidation [at methionine, histidine or tryptophan residues (+15.995 Da)], acetylation (+42.011 Da), amidation (-0.984 Da), phosphorylation [at serine, threonine or tyrosine residues
Unique expressed proteins, identified for every individual donor, with and without HNP1 treatment, were uploaded and analyzed with the GeneGo pathway maps and process networks tools of MetaCoreTM, Version 22.1 build 70,800 (GeneGo, St. Jospeh, MI, USA).
All data are presented as the means ± SEM, performed in GraphPad Prism 9.2 software. Mann-Whitney U tests were performed in R Software.
ELISA analysis
In a last step, to validate the proteomic results, the presence of 7 cytokines was measured in the supernatants of the same cocultures, as described earlier, and an additional donor with a U-PLEX proinflammatory human kit, according to the manufacturer’s protocol [Meso Scale Discovery (MSD, Rockville, MD, USA)]. The cytokine panel existed of TNF-α, IL-1β, IL-2, IL-4, IL-6, IL-12p70 and IL-18. CXCL8 was measured in the supernatants of A549/PBMC cocultures of four other donors with an in-house developed ELISA[13].
RESULTS
Proteomic results for three healthy donors
Mass spectrometry analyses were run separately for the secretome and the proteomic cell content of in vitro cocultures of A549 cells plus peripheral blood mononuclear cells (PBMCs) from healthy donors. The coculture of every donor was tested with HNP1 stimulation and no stimulation (PBS control). PEAKS software (Bioinformatics Solutions Inc., Waterloo, ON, Canada) was used to identify peptides and corresponding proteins. Afterwards, for each corresponding donor, the identified proteins per condition were pooled for both the secretome and the cell content. Sequentially, the identified protein list was filtered for proteins unique to only the HNP1-stimulated condition (i.e., absent in cocultures without HNP1 stimulation). Using these lists of unique proteins for each individual donor in a MetaCore analysis, different immune-related pathways and process networks appear to be upregulated following HNP1 stimulation
Figure 3. Overview of immune-related pathway maps and process networks in in vitro cocultures of A549 and PBMCs from three healthy donors, with (orange) and without (blue) treatment with human neutrophil peptide 1 (HNP1), after proteomic and MetaCoreTM analyses. A distinction is made between common pathway maps and process networks observed in all three healthy donors (A) and those observed in two out of three healthy donors (B). Significant differences (P ≤ 0.05) were retained after the Mann-Whitney U test, performed in R Software: P = 0.05 for all nine upregulated pathways for all three donors (A).
The proteomic results of A549 cells cocultured in vitro with PBMCs show nine immune-related pathways that are clearly upregulated after HNP1 stimulation for all three donors (represented in Figure 3A) and nine additional pathways in two out of three donors [Figure 3B]. Furthermore, the top network objects ranked by occurrence in the most frequently identified pathways and process networks are also listed by MetaCoreTM. The summarized network objects, observed in at least two out of the three donors, are presented in Table 1.
Unique network objects, according to MetaCoreTM analysis, that are expressed in at least 2 out of 3 donors for either HNP1 stimulation or without stimulation of the in vitro A549/PBMC coculture
No stimulation | HNP1 stimulation |
MyHC | IP3 receptor Calcineurin A (catalytic) MEK1/2 MEK1(MAP2K1) NF-kB MEK2(MAP2K2) PKC RelA (p65 NF-kB subunit) Lyn CCL2 cPKC (conventional) Btk NF-AT1(NFATC2) PREX1 PP2A catalytic p300 PP2A regulatory p27KIP1 PGES NF-kB p65/p65 Calcineurin A (catalytic) |
Stable isotope labeling with amino acids in cell culture (SILAC)
SILAC labeling of specific cell types has mainly the purpose of quantitative analyses, but we have used this approach to trace the cell origin of the identified proteins. This approach was useful for the analysis of amphoterin/high mobility group box 1 protein (HMGB1). HMGB1 can be secreted from different types of immune cells, but it can also passively be released from necrotic or damaged cells to serve as an alarmin or damage-associated molecular pattern (DAMP). From Figure 3A, it can be observed that amphoterin signaling is upregulated after HNP1 stimulation of the in vitro coculture. By applying SILAC labeling of the A549 cells, we could trace the origin of the detected HMGB1. Both SILAC-labeled and unlabeled peptides corresponding to HMGB1 were detected [Table 2]. The unlabeled peptides corresponding to HMGB1 can originate from the immune cells or can be newly synthesized by the tumor cells during coculturing, as the coculture medium did not contain labeled amino acids. The presence of SILAC-labeled peptides [Table 2] does, however, indicate with certainty the passive release of HMGB1 by (damaged) tumor cells.
Identification of HMGB1 peptides (SILAC labeled/unlabeled) in the A549/PBMC cocultures
Peptide of HMGB1 | SILAC peptide of HMGB1 |
YEKDIAAYR | YEK(+6.02)DIAAYR(+10.01) |
TYIPPKGETKK | TYIPPK(+6.02)GETK(+6.02) |
TYIPPKGETK | |
RPPSAFFLFC(+57.02)SEYRPK(-0.98) | |
RPPSAFFLFC(+57.02)SEYRPK | |
MSSYAFFVQTC(+57.02)REEHK | MSSYAFFVQTC(+57.02)R(+10.01) |
MSSYAFFVQTC(+57.02)REEHKK | |
MSSYAFFVQTC(+57.02)R | |
M(+15.99)SSYAFFVQTC(+57.02)R | |
LKEKYEKDIAAYR | |
LKEKYEK | |
LGEMWNNTAADDKQPYEKK | LGEMWNNTAADDK(+6.02)QPYEK(+6.02) LGEMWNNTAADDK(+6.02)QPYEK LGEMWNNTAADDK(+6.02) |
LGEMWNNTAADDKQPYEK | |
LGEMWNNTAADDK | |
LGEMW(+15.99)NNTAADDKQPYEK | |
LGEM(+15.99)WNNTAADDKQPYEKK | |
LGEM(+15.99)WNNTAADDKQPYEK | |
KLGEMWNNTAADDKQPYEK(-0.98) | |
KLGEMWNNTAADDKQPYEK | |
KLGEMWNNTAADDK | |
KLGEMW(+15.99)NNTAADDKQPYEKK | |
KLGEMW(+15.99)NNTAADDKQPYEK | |
KLGEM(+15.99)WNNTAADDKQPYEKK | |
KLGEM(+15.99)WNNTAADDKQPYEK | |
KKHPDASVNFSEFSK | K(+6.02)HPDASVNFSEFSK(+6.02) |
KHPDASVNFSEFSKK | |
KHPDASVNFSEFSK | |
HPDASVNFSEFSKK | |
HPDASVNFSEFSK | |
IKGEHPGLSIGDVAKK | IK(+6.02)GEHPGLSIGDVAK(+6.02) |
IKGEHPGLSIGDVAK | |
GKFEDMAK | GK(+6.02)FEDMAK(+6.02) |
GKFEDM(+15.99)AK | |
EKGKFEDMAK | |
GEHPGLSIGDVAK | GEHPGLSIGDVAK(+6.02) |
FKDPNAPK | FK(+6.02)DPNAPK(+6.02) |
Confirmation of upregulated immune-related pathways by multiplex ELISA
Multiplex ELISA analysis was performed on the same cocultures, as described earlier, and an additional donor with a U-PLEX (MSD, Rockville, MD, USA). Antibodies were specific for the following 7 cytokines: TNF-α, IL-1β, IL-2, IL-4, IL-6, IL-12p70 and IL-18. The rationale behind this proinflammatory cytokine panel is to confirm the upregulated pathways observed with proteomics, as for most of them, the most important cytokine(s), such as IL-1β, IL-2 and IL-6 [Figure 3], were not observed in the proteomics data set. The inhibiting cytokine IL-4 was not detected in any condition. The detected concentrations (mean ± SEM) of the six other proinflammatory cytokines are presented in Figure 4A and Table 3. The proinflammatory chemokine CXCL8 was measured in the supernatants of A549/PBMC cocultures of four other donors with ELISA and the detected concentrations (means ± SEM) are presented in Figure 4B and Table 3.
Figure 4. Cytokine secretion in the supernatants of in vitro cocultures of A549 and PBMCs from healthy donors, with (orange) and without (blue) treatment with human neutrophil peptide1 (HNP1). The results are presented as means ± SEM of the different donors
Means ± SEM for every cytokine analyzed with multiplex ELISA, performed in GraphPad Prism 9.2 software
Cytokine | Control | HNP1 stimulation | ||
Mean | SEM | Mean | SEM | |
IL-1β | 62.000 | 3.789 | 236.725 | 95.756 |
IL-2 | 122.600 | 32.437 | 162.700 | 17.778 |
IL-6 | 263.875 | 103.550 | 13,648.250 | 6,299.793 |
IL-18 | 146.800 | 36.151 | 175.250 | 51.930 |
IL-12p70 | 26.335 | 6.057 | 37.400 | 1.445 |
TNF-α | 63.325 | 17.341 | 688.625 | 403.035 |
CXCL8 | 3.050 | 1.413 | 140.050 | 24.517 |
DISCUSSION
Proteomic approaches, including mass spectrometry and protein array analysis, have already revealed important insights into underlying molecular signaling pathways in ovarian cancer research. This may facilitate the choice of therapy to better overcome therapy resistance and improve the patient’s survival outcome[14]. In this study, the upregulated immune-related pathways [Figure 3] in the context of HNP1 stimulation are further discussed, demonstrating the feasibility of proteomic approaches to provide a better understanding of the mechanisms through which HNP1 acts in a cancer-related context. Upregulated pathways were further validated using a U-PLEX proinflammatory human cytokine kit from MSD [Figure 4].
Upregulation of the IFN-γ signaling pathway can be observed in Figure 3B, which was already confirmed by increased IFN-γ concentrations in HNP1-stimulated cocultures in our previous study[6], which formed the basis for this study. Another important cytokine signaling pathway, IL-6 signaling, showed an evident upregulation after treatment of the coculture with HNP1 [Figure 3A] and was validated by a significant increase of the IL-6 cytokine in the HNP1-treated cocultures [Figure 4A]. IL-6 is produced by many cell types and has a wide spectrum of biological activities. IL-6 is important to bridge the innate and adaptive immune response, as many innate immune cells produce and respond to IL-6. Furthermore, IL-6 is known for its regulatory role in T cell differentiation and activation and is therefore also involved in adaptive immune responses[15,16]. Its pleiotropic effects will become clear throughout the following discussion.
Triggering receptor expressed on myeloid cells (TREM1) signaling is clearly upregulated after human neutrophil peptide 1 (HNP1) treatment of the coculture. TREM1 is expressed on myeloid cells, i.e., monocytes, macrophages and granulocytes. After activation of this receptor, proinflammatory innate immune responses are amplified, especially through synergism with TLR signaling[17,18]. Bouchon et al. reported that activation of the TREM1 receptor on monocytes was associated with increased production of TNF-α and IL-1β (both confirmed to be upregulated, see Figure 4A). Also, TREM1 receptor activation is known to result in higher concentrations of monocyte chemoattractant protein 1 [MCP-1, also referred to as chemokine (C-C motif) ligand 2 (CCL2)][19]. CCL2 was indeed exclusively present in the secretome of HNP1-stimulated cocultures [Table 1]. Next, several proteins inducing leucocyte chemotaxis, more precisely chemokines, are observed to a higher extent after HNP1 treatment of the coculture. Chemokine expression is necessary for a proper inflammatory response by enabling extravasation and activation of the PBMCs and neutrophils from the blood into the surrounding tissues. CCL2 [Table 1] and its receptor CCR2 are associated with T cell and monocyte attraction and further empower the phagocytic and killing ability of monocytes[20]. We detected increased levels of the most potent human neutrophil chemoattractant/activator interleukin-8 (IL-8/CXCL8) by specific ELISA and pathway analysis in cocultures of A549/PBMCs (neutrophil activation, see Figures 3B and 4B). Downstream of the chemokine receptors, PKC and IP3 receptor signaling is activated[21]. Components of those signaling pathways were only detected in HNP1-stimulated in vitro cocultures [Table 2]. Complementary to the induction of leucocyte chemotaxis, the PLAU signaling pathway is also upregulated following the HNP1 treatment [Figure 3B]. The central component of this pathway is the plasminogen activator, urokinase (PLAU), which, after binding to the urokinase receptor (uPAR), mediates a variety of functions. These functions include vascular homeostasis, inflammation and tissue repair[22]. In particular, PLAU plays an essential role in cytoskeletal regulation, cell adhesion and migration[23]. It has been shown that remodeling of the cytoskeleton is involved in the recruitment of neutrophils[24]. Also, PLAU is an important mediator for the conversion of plasminogen to plasmin and upregulation of this plasmin signaling pathway is also observed [Figure 3A]. The extracellular protease plasmin is capable of releasing intracellular signals responsible for fibrin degradation and matrix turnover, both important during cell migration and cell invasion. Moreover, it has been shown in in vivo mice studies that plasmin mediates mononuclear cell recruitment through activation of the MEK/ERK/NF-kB pathway, eventually leading to CCL2 release[25]. Both CCL2, three MEK and two NF-kB-related proteins were specifically expressed after stimulation of the cocultures with HNP1 [Table 1]. We also observed upregulation of the IL-1 signaling pathway in two (from the three tested donors) of the in vitro cocultures after HNP1 treatment. Upregulation of IL-1-type cytokines (including IL-1β and IL-18, see Figure 4A) launches innate immune reactions by induction of CXCL8, TNF-α and IL-6 (both upregulated in Figure 4) and CCL2, what we confirm in Table 1[26].
Next, the tyrosine kinase Lyn is observed in HNP1-stimulated cocultures [Table 1] and the Lyn-mediated cytokine production pathway is upregulated in all three tested donors after treatment with HNP1 [Figure 3A]. Lyn mainly functions through the phosphorylation of inhibitory receptors, resulting in the inhibition of different signaling pathways[27]. In contrast, Lyn has a predominant role in B cell development and differentiation and is thought to play a positive regulatory role in LPS-induced dendritic cell (DC) maturation[27]. Krebs et al. proved in a mouse model that Lyn enhances endotoxin-induced DC maturation[28] in response to an increased IFN-γ production (proven in our previous study[6]) by natural killer (NK) cells[28]. The IFN-activated DCs, in turn, produce IL-1β, IL-6, IL-12, IL-18 and TNF-α, for which upregulation in our cocultures was confirmed by multiplex ELISA [Figure 4A]. Upregulation of NK cell cytotoxicity was observed upon HNP1 stimulation of cocultures [Figure 3B]. IL-12 and IL-18 are implicated in NK cell activation, together with IL-2 and all three cytokines also slightly increased in expression after HNP1 stimulation [Figure 4A][28,29]. DCs activated by IFN-γ produce more cytokines, but IFN-stimulated DCs and macrophages also phagocytose [Figure 3A] more effectively, enhancing uptake and processing of, in our experimental context, dying tumor cells for antigen presentation. Antigen presentation, combined with cytokine production, causes the activation of adaptive immune cells and provides the bridge between innate and adaptive immunity[30,31]. DC activation and maturation is also driven by IL-1 and TNF-α, both confirmed to be upregulated after HNP1 stimulation [Figures 3B and 4A]. In addition, we detected the upregulation of histamine signaling in DCs after treatment of the coculture with HNP1 [Figure 3B]. This pathway promotes the migration of immature DCs, supports normal differentiation of the DCs and stimulates antigen uptake and presentation through cross-presentation[32]. As histamine is typically linked with mast cell activation and allergy, thus IgE production, MetaCore also listed IgE signaling [Figure 3B]. However, we see no obvious function for the IgE pathway in our coculture system, nor in a tumor microenvironment.
Amphoterin/HMGB1 is highly present in the nucleus of different cell types and acts as a potent activator of inflammation when it is released extracellularly[30,33]. An upregulation of amphoterin signaling is observed in Figure 3A after HNP1 stimulation of the in vitro coculture. HMGB1 can be secreted from different types of immune cells, including dendritic cells, macrophages, monocytes and NK cells, but it can also passively be released from necrotic or damaged cells to serve as an alarmin or damage-associated molecular pattern (DAMP). These molecules can act through Toll-like receptors (TLRs) and the receptor for advanced glycation end products (RAGE) for activation of innate immune responses[34]. By using SILAC labeling of the A549 cells, we could demonstrate that part of the detected HMGB1 is passively released by damaged tumor cells [Table 2], which can indicate necrosis of the tumor cells in the in vitro coculture after HNP1 stimulation. Indeed, we observed reduced tumor cell numbers in cocultures of NSCLC cell lines and PBMCs in the presence of HNP1 compared to untreated cocultures[6]. Activation of the adaptive immune cells in our cocultures is probably rather aspecific, through the production of lymphocyte-activating cytokines (e.g., IL-1, IL-2, IL-6) released by innate cells upon encountering DAMPs (e.g., HMGB1) set-free by the dying tumor cells. We believe that this is the reason why several adaptive immune pathways (BCR signaling, TCR signaling, T helper cell differentiation) are picked by the enrichment analysis. However, in NSCLC patients responding to ICI[6], adaptive immunity can be supported by the presence of HNPs in the tumor microenvironment through the empowerment of innate immunity.
Thus, HNP1 seems to be an important mediator in linking the innate and adaptive immune systems, but the immune-enhancing properties of HNPs are not yet fully unraveled. In future studies, the identified effected immune-related pathways can be examined in more detail.
We can conclude that performing proteomic approaches, including pathway analyses, are a powerful tool to provide insights into biological systems. By doing so, we obtained a better understanding of the biological activity of the human neutrophil peptide 1 (HNP1) in in vitro A549/PBMC cocultures, as a simplified model for the tumor microenvironment of NSCLC. From these results, it can be concluded that treatment with HNP1 of the in vitro cocultures initiates a cascade of inflammatory pathways, supporting leucocyte recruitment, activation (e.g., cytokine secretion), DC maturation, phagocytosis and antigen presentation. Improved antigen presentation, in turn, would allow more efficient adaptive anti-tumoral immunity in patients. As the PBMCs in the cocultures are derived from healthy donors, the reduced tumor cell numbers in cocultures of NSCLC cell lines and PBMCs in the presence of HNP1 can be expected to be rather a consequence of innate immune activation.
DECLARATIONS
AcknowledgmentsTechnical assistance for (multiplex) ELISA analysis by An Jacobs and Noëmie Pörtner was highly appreciated.
Authors’ ContributionsMade substantial contributions to the conception and design of the study and interpretation: Berghmans E, Smits E, Struyf S, Baggerman G
Performed data acquisition; Flender D, Berghmans E
Performed data analysis and drafted the work: Berghmans E
Availability of Data and MaterialsThe proteomics data presented in this study are available on request from the corresponding author. The data are not publicly available due to GDPR regulations.
Financial Support and SponsorshipNone.
Conflicts of InterestAll authors declared that there are no conflicts of interest.
Ethical Approval and Consent to ParticipateThe study was approved by the Ethics Committee of the University of Antwerp, reference number 15/48/517. Informed consent was obtained from all subjects involved in the study to publish this paper.
Consent for PublicationBuffy coats derived from human whole blood donations, voluntarily provided by three healthy adult donors, were supplied by the Red Cross Flanders Blood Service (Mechelen, Belgium). All donors provided written consent for research and publication.
Copyright© The Author(s) 2023.
Supplementary MaterialsREFERENCES
1. World Health Organization. Cancer today. In: International Agency for Research on Cancer; 2022. Available from: https://gco.iarc.fr/today/home [Last accessed on 29 March 2022].
2. Bai R, Lv Z, Xu D, Cui J. Predictive biomarkers for cancer immunotherapy with immune checkpoint inhibitors. Biomark Res 2020;8:34.
3. Robert C, Long GV, Brady B, et al. Nivolumab in previously untreated melanoma without BRAF mutation. N Engl J Med 2015;372:320-30.
4. Daud AI, Wolchok JD, Robert C, et al. Programmed death-ligand 1 expression and response to the anti-programmed death 1 antibody pembrolizumab in melanoma. J Clin Oncol 2016;34:4102-9.
5. Moschetta M, Uccello M, Kasenda B, et al. Dynamics of neutrophils-to-lymphocyte ratio predict outcomes of PD-1/PD-L1 blockade. Biomed Res Int 2017;2017:1506824.
6. Berghmans E, Jacobs J, Deben C, et al. Mass spectrometry imaging reveals neutrophil defensins as additional biomarkers for anti-PD-(L)1 immunotherapy response in NSCLC patients. Cancers 2020;12:863.
7. Berghmans E, Baggerman G. Mass spectrometry (imaging) for detection and identification of cyclic AMPs: focus on human neutrophil peptides (HNPs). In: Enany S, Masso-silva J, Savitskaya A, editors. Insights on Antimicrobial Peptides. IntechOpen; 2021.
9. Zeng X, Yang P, Chen B, et al. Quantitative secretome analysis reveals the interactions between epithelia and tumor cells by in vitro modulating colon cancer microenvironment. J Proteomics 2013;89:51-70.
10. Chen CY, Chi LM, Chi HC, et al. Stable isotope labeling with amino acids in cell culture (SILAC)-based quantitative proteomics study of a thyroid hormone-regulated secretome in human hepatoma cells. Mol Cell Proteomics 2012;11:M111.011270.
11. Zougman A, Selby PJ, Banks RE. Suspension trapping (STrap) sample preparation method for bottom-up proteomics analysis. Proteomics 2014;14:1006-0.
12. Meier F, Brunner AD, Koch S, et al. Online parallel accumulation-serial fragmentation (PASEF) with a novel trapped ion mobility mass spectrometer. Mol Cell Proteomics 2018;17:2534-45.
13. Cockx M, Blanter M, Gouwy M, et al. The antimicrobial activity of peripheral blood neutrophils is altered in patients with primary ciliary dyskinesia. Int J Mol Sci 2021;22:6172.
14. Ghose A, Gullapalli SVN, Chohan N, et al. Applications of proteomics in ovarian cancer: dawn of a new era. Proteomes 2022;10:16.
15. Choy E, Rose-john S. Interleukin-6 as a multifunctional regulator: inflammation, immune response, and fibrosis. J Scleroderma Relat Disord 2017;2:S1-5.
16. Neurath MF, Finotto S. IL-6 signaling in autoimmunity, chronic inflammation and inflammation-associated cancer. Cytokine Growth Factor Rev 2011;22:83-9.
17. Roe K, Gibot S, Verma S. Triggering receptor expressed on myeloid cells-1 (TREM-1): a new player in antiviral immunity? Front Microbiol 2014;5:627.
18. Tammaro A, Derive M, Gibot S, Leemans JC, Florquin S, Dessing MC. TREM-1 and its potential ligands in non-infectious diseases: from biology to clinical perspectives. Pharmacol Ther 2017;177:81-95.
19. Bouchon A, Dietrich J, Colonna M. Cutting edge: inflammatory responses can be triggered by TREM-1, a novel receptor expressed on neutrophils and monocytes. J Immunol 2000;164:4991-5.
20. Adams DH, Lloyd AR. Chemokines: leucocyte recruitment and activation cytokines. Lancet 1997;349:490-5.
22. Stepanova VV, Tkachuk VA. Urokinase as a multidomain protein and polyfunctional cell regulator. Biochemistry 2002;67:109-18.
23. Cesarman-Maus G, Hajjar KA. Molecular mechanisms of fibrinolysis. Br J Haematol 2005;129:307-21.
24. Kobayashi SD, DeLeo FR. Role of neutrophils in innate immunity: a systems biology-level approach. Syst Biol Med 2009;1:309-33.
25. Carmo AA, Costa BR, Vago JP, et al. Plasmin induces in vivo monocyte recruitment through protease-activated receptor-1-, MEK/ERK-, and CCR2-mediated signaling. J Immunol 2014;193:3654-63.
27. Xu Y, Harder KW, Huntington ND, Hibbs ML, Tarlinton DM. Lyn tyrosine kinase: accentuating the positive and the negative. Immunity 2005;22:9-18.
28. Krebs DL, Chehal MK, Sio A, et al. Lyn-dependent signaling regulates the innate immune response by controlling dendritic cell activation of NK cells. J Immunol 2012;188:5094-105.
29. Paul S, Lal G. The molecular mechanism of natural killer cells function and its importance in cancer immunotherapy. Front Immunol 2017;8:1124.
30. Blanco P, Palucka AK, Pascual V, Banchereau J. Dendritic cells and cytokines in human inflammatory and autoimmune diseases. Cytokine Growth Factor Rev 2008;19:41-52.
31. Fu YL, Harrison RE. Microbial phagocytic receptors and their potential involvement in cytokine induction in macrophages. Front Immunol 2021;12:662063.
32. Amaral MM, Davio C, Ceballos A, et al. Histamine improves antigen uptake and cross-presentation by dendritic cells. J Immunol 2007;179:3425-33.
33. Magna M, Pisetsky DS. The role of HMGB1 in the pathogenesis of inflammatory and autoimmune diseases. Mol Med 2014;20:138-46.
Cite This Article
Export citation file: BibTeX | RIS
OAE Style
Berghmans E, Flender D, Smits E, Struyf S, Baggerman G. A proteomic approach to better understand the role of human neutrophil peptides in the NSCLC microenvironment. J Cancer Metastasis Treat 2023;9:8. http://dx.doi.org/10.20517/2394-4722.2022.116
AMA Style
Berghmans E, Flender D, Smits E, Struyf S, Baggerman G. A proteomic approach to better understand the role of human neutrophil peptides in the NSCLC microenvironment. Journal of Cancer Metastasis and Treatment. 2023; 9: 8. http://dx.doi.org/10.20517/2394-4722.2022.116
Chicago/Turabian Style
Berghmans, Eline, Daniel Flender, Evelien Smits, Sofie Struyf, Geert Baggerman. 2023. "A proteomic approach to better understand the role of human neutrophil peptides in the NSCLC microenvironment" Journal of Cancer Metastasis and Treatment. 9: 8. http://dx.doi.org/10.20517/2394-4722.2022.116
ACS Style
Berghmans, E.; Flender D.; Smits E.; Struyf S.; Baggerman G. A proteomic approach to better understand the role of human neutrophil peptides in the NSCLC microenvironment. J. Cancer. Metastasis. Treat. 2023, 9, 8. http://dx.doi.org/10.20517/2394-4722.2022.116
About This Article
Copyright
Data & Comments
Data
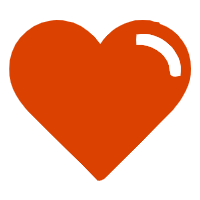

Comments
Comments must be written in English. Spam, offensive content, impersonation, and private information will not be permitted. If any comment is reported and identified as inappropriate content by OAE staff, the comment will be removed without notice. If you have any queries or need any help, please contact us at support@oaepublish.com.