Imaging traumatic facial nerve injuries: a narrative review of current strategies and future directions for cranial nerve imaging
Abstract
Traumatic facial nerve injuries can result in temporary or permanent loss of function. Restoration of facial expression may occur spontaneously or require surgical intervention. Although thorough examination and history can localize the site of facial nerve damage, it can be difficult to predict if and when recovery will occur. This is salient because the window for optimal outcomes from surgical re-neurotization can be as short as 1 to 2 years, after which functional loss may be irreversible. It is essential to offer patients the most appropriate treatment plan based on prognosis, and imaging plays an essential role in localizing the site and morphology of nerve injury. Multiple imaging modalities have been used to evaluate the facial nerve, including computed tomography (CT) and, more recently, advanced magnetic resonance imaging (MRI) and ultrasound (US). CT and MRI are more commonly implemented; however, Diffusion tensor tractography, high-resolution US, and functional US are gaining traction for studying cranial nerve pathology. Until recently, the morphology of facial nerve and other cranial nerve injuries could only be inferred using non-invasive diagnostic techniques. With the advent of newer imaging technologies and techniques to examine nerves, more refined assessment and prognostic information is now possible. This article reviews up-to-date cranial nerve imaging techniques from the last ten years and explores future avenues for facial nerve imaging.
Keywords
INTRODUCTION
The paired facial nerves play an essential role in facial muscle movement. Injury can have devastating consequences, including corneal exposure keratopathy, obstructed gaze, nasal obstruction, loss of oral competence, impaired speech and gustation, and loss of social facial expression. Traumatic facial palsy is a distinct clinical entity with a wide range of causes and manifestations. This review will summarize current and emerging cranial nerve imaging strategies that can be implemented for the diagnosis and treatment of traumatic facial nerve injuries.
The Seddon or Sunderland classification systems are widely used to classify peripheral nerve injuries and are useful to help predict the likelihood of spontaneous recovery[1]. The House-Brackman scale is often employed to characterize the degree of facial nerve dysfunction and track recovery over time[2]. Patient history and findings over serial examination guide the treatment approach. It is essential to determine if reinnervation procedures may be necessary before muscular atrophy precludes this reconstructive approach.
The magnitude of injury and the time interval to regeneration or repair influence the degree of peripheral nerve injury recovery. Axon regeneration is influenced by the distance that must be traversed to re-innervate motor and sensory targets[3]. Longer distances require a longer time to regenerate[4]. Furthermore, chronic denervation negatively impacts Schwann cell recovery in the denervated target muscles. Over time, the chronicity of injury results in diminished recovery potential[5]. Therefore, optimal reinnervation of muscles requires prompt diagnosis and earlier intervention[6]. For injuries that are not diagnosed promptly or are not amenable to immediate repair, reinnervation procedures may be more successful if performed within 12 to 18 months of injury[7]. After this timeframe, the likelihood of optimal recovery diminishes significantly due to chronic denervation and muscle atrophy[8]. By harnessing high-resolution imaging and advanced image processing techniques, it may be possible to identify and track the degree of nerve injury and recovery sooner and more reliably, thus improving outcomes for patients.
Anatomy
The facial nerve is a paired cranial nerve with sensory, parasympathetic, and motor components. It originates from the central nervous system as a mixed nerve from three midbrain nuclei. Upper motor neurons from the corticobulbar tract pass into the facial motor nucleus within the pons. From here, the lower motor neurons innervate the ipsilateral hemiface. The forehead is unique in receiving innervation from both cerebral cortices. As it arises from the brainstem and enters the petrous portion of the temporal bone, it traverses in a serpentine fashion before exiting at the stylomastoid foramen[9]. This tortuous intra-temporal course renders the nerve susceptible to compression due to swelling, or laceration from osseous fragments.
After exiting the stylomastoid foramen and giving off several motor and sensory branches, it courses towards the parotid gland, where it divides into two major trunks: the superior temporofacial trunk and the inferior cervicofacial trunk. These trunks further arborize to form the temporal, zygomatic, buccal, mandibular, and cervical branches. The temporal, mandibular, and cervical branches typically have few interconnections, but the zygomatic and buccal often have a vast network of connections that provide redundant innervation of the midface.
The location of traumatic insult will result in specific functional deficits. In addition to physical examination and electrodiagnostic studies, imaging is an important adjunctive tool to help pinpoint traumatic facial nerve injuries.
Trauma mechanisms to the facial nerve
Traumatic facial nerve injury may account for up to 10% of the causes of facial nerve palsy[10]. Trauma can be broadly classified as blunt or penetrating. Blunt injuries typically arise from direct blows, which generate acceleration-deceleration forces applied to the intra-temporal course, or result in temporal bone fractures that lacerate the intra-temporal facial nerve. Edema of the nerve and surrounding vaso nervorum can result in secondary ischemic compression and associated facial nerve palsy[11]. Less common causes of blunt trauma include birth trauma or barotrauma from exposure to high altitudes or while SCUBA diving[12]. Penetrating trauma can be sharp, such as knife or shrapnel injuries, or explosive, as with gunshot injuries. These injuries result in nerve transection, often of the extratemporal facial nerve[13]. A full history and physical exam that details the mechanism of injury, pre-morbid facial nerve function and associated symptoms must be documented. Electrodiagnostic studies are sensitive and specific and are often obtained to help diagnose and monitor nerve injury. These studies are time-intensive, invasive procedures that sometimes require a waiting period of weeks after injury for reliable information, but can offer valuable information to corroborate imaging findings.
Imaging modalities
Multiple imaging modalities are available to evaluate the facial nerve, including computed tomography (CT), magnetic resonance imaging (MRI), and ultrasound (US). Historically, the modality chosen was related to the suspected area of injury, with high-resolution CT commonly employed for intra-temporal fallopian canal injuries and contrast-enhanced MRI employed for brainstem lesions or injuries. MRI is also the imaging modality of choice to visualize the entire course of the facial nerve. Accurate prognosis of functional nerve recovery remains difficult to predict, and currently, there is limited data on imaging findings specific to facial nerve trauma. Nevertheless, recent developments in MRI and US capabilities have expanded the role these modalities will likely play in the future.
CT imaging is often obtained during trauma evaluation and can identify osseous injuries around the intra-temporal segment of the facial nerve. Temporal bone injuries are best discerned with this imaging modality, with dedicated temporal bone CT scans offering higher specificity for fractures compared to Magnetic Resonance Imaging (MRI)[14]. MRI can provide highly detailed images of soft tissues, with specialized sequences offering high-resolution detail that is important for discerning nerve injuries. This modality is limited by higher costs and the time and logistics required for acquisition.
More recently, MRI-based diffusion tensor tractography (DTT) is a technique that has been used to create three-dimensional (3D) reconstructions of nerve structures. It has demonstrated the ability to identify the displacement of cranial nerve fibers caused by tumors. Although its application in evaluating traumatic facial nerve injuries has not been described yet, it presents an exciting emerging avenue for nerve imaging.
Neither CT nor MRI imaging can be performed outside of dedicated imaging centers. The development of specialized US techniques that create high-resolution images has opened up new possibilities for nerve imaging. Advantages of ultrasound imaging include lower relative cost, point-of-care availability, non-invasive data acquisition, and the ability to perform it serially at office visits.
Computed tomography
CT imaging can reveal gross abnormalities of the facial nerve, such as transection or avulsion, and remains a cost-effective method for rapidly assessing critical structures that may. It offers minimal direct information about the nerve itself, however.
Initial trauma scans performed at trauma centers are designed to identify gross pathology with immediate life-threatening impact, and are not of sufficient quality to discern the intricate structures associated with the facial nerve. Patients suspected of having temporal bone fractures often undergo dedicated High-resolution CT (HRCT) to obtain finer detail, a technique that has been well-established[15,16]. This is performed with tomographic slices of 1 mm or less, and post-processed with a specialized bone algorithm. Gupta et al. describe a protocol of non-contrast HRCT with 0.3 mm axial slices through both temporal bones, as well as 0.6 mm coronal sections and Poschl reformats, with dose settings of 120 kVp and CTDI volume of 55.5-62.6 and 140-220 mAs[15]. Mu et al. described a correlation between enlarged geniculate ganglion fossa and fracture of the temporal bone in this region in patients with traumatic facial palsy[14].
Common sites of injury are at the geniculate ganglion or at the mastoid segment of the facial canal. Longitudinal temporal bone fractures, defined by the relationship of the long axis relative to the petrous portion, are the most common fracture type. Transverse fractures are more commonly associated with facial nerve palsy[10]. Proximal injuries involving the geniculate ganglion, tympanic and labyrinthine segments of the temporal bone can reliably predict damage to the facial nerve, but often underestimate injury at the distal mastoid segment[17,18].
Magnetic resonance imaging
Standard T1 and T2-weighted MRI protocols are helpful to localize areas within the head and neck with suspected injury. The facial nerve is comprised of high fat content white matter fibers surrounded by high water content cerebrospinal fluid (CSF); therefore, some neuroradiologists recommend obtaining T2-weighted sequences including fast imaging employing steady-state acquisition (FIESTA) and constructive interference in steady-state (CISS) for suspected facial nerve injury. These sequences permit improved resolution of the nerve, similar to contrast myelography[19]. Facial nerve imaging protocols often include these sequences, as well as post-contrast fluid-attenuated inversion recovery (FLAIR) and T1-weighted sequences, to aid in subtracting CSF signal for improved delineation of the nerve[9,20].
To assess the extracranial portion of the facial nerve as it courses through the parotid gland, three-dimensional reconstruction of reversed fast imaging steady-state precession diffusion-weighted (3D-PSIF DWI) with a microsurface coil has been shown to provide high-detail images capable of delineating nerve branches when using a 3 Tesla (3T) MRI machine[21].
An exciting set of specialized MRI techniques gaining interest in peripheral nerve imaging are diffusion weighted imaging (DWI) and diffusion tensor imaging (DTI). The anisotropic, or direction-dependent, nature of axonal fascicles results in final post-processed images that can show the course of these nerve fascicles. DTI is post-processing mathematical modeling that harnesses this differential diffusion effect. Three-dimensional reconstructions of the DWI/DTI imaging protocol visually depict the neural tracts, which is referred to as Diffusion Tensor Tractography (DTT). This technique can be particularly useful for mapping the facial nerve, and has been shown to successfully visualize the nerve from the pons to the internal auditory meatus[22,23]. More recently, DTT has been used for preoperative identification of the facial nerve during vestibular schwannoma resection. Li et al. demonstrated that DTT was able to correctly define the facial nerve course in 17 of 18 patients, confirmed intraoperatively with neuronavigation and evoked potentials with facial nerve monitoring [Figures 1 and 2][24]. Savardekar et al. published a systematic review that identified 14 studies with 234 patients who underwent preoperative DTT imaging to identify the facial nerve course prior to vestibular schwannoma resection[23]. DTT was shown to have 87.1% concordance with intraoperative findings (205 patients); in 18 patients, the DTT was verified but discordant (7.6%), and in 11 patients, the facial nerve could not be identified or verified (4.7%). As more experience is gained with this technique, automation and uniformity of data processing will likely lead to improvements and clinical utility[25].
Figure 1. Preoperative Diffusion Tensor Tractography in a patient with unilateral facial nerve schwannoma, showing the course of the facial nerve along the anterior middle third of the tumor. (A-C) The axial, coronal, and sagittal view of diffusion tensor tractography showing the facial nerve tract (yellow arrow), the tumor (T) and the contralateral normal facial nerve tract (blue arrow). (D) The three-dimensional model of the facial nerve tract (yellow arrow), the tumor (T) and the contralateral normal facial nerve tract (blue arrow). L: left; R: right. With permission from Chen et al.[18].
Figure 2. MRI and DTI images showing the facial nerve (cranial nerve VII, CN VII) and the vestibular-cochlear nerve (cranial nerve VIII, CN VIII) at the cerebellopontine angle. A unilateral vestibular schwannoma (VS) grade I is visualized on standard (A) and high-resolution T2 sequences (B). (C) A tensor map is generated in multiple axes and high-resolution diffusion tensor data is generated. Tensors were suppressed below a predetermined threshold. (D) The high-resolution images were then processed to generate tractography images of CN VII and CN VIII. (E) The improved resolution between these two cranial nerves is seen in the panel. With permission from Epprecht et al.[27].
Recently, DTT was performed on a patient who sustained traumatic flexion-hyperextension, and suggested unilateral discontinuity of the trigeminal nerve, indicating that DTI may be useful in the setting of traumatic facial nerve injury[26]. DTT is resource intensive, requiring at least a 1.5T MRI scanner and dedicated computational experts to review the data and generate the reconstructions. Nevertheless, continued development over the last decade has demonstrated its promise in imaging nerves outside the central nervous system and may be a useful adjunct to diagnose and monitor facial nerve injuries in the future.
Ultrasound
Conventional ultrasound is a well-established technique with recent noteworthy advancements. Standard ultrasonography for nerves or blood vessels utilizes linear or phased array transducers over frequencies between 2 MHz and 15 MHz. In medical applications, high-frequency ultrasound (HFUS) uses transducers between 15 MHz and 30 MHz, while ultra-high frequency ultrasound (UHFUS) transducers are relatively new, and can operate at frequencies up to 100 MHz, generating images with a resolution of up to
The extratemporal facial nerve arborizes within the parotid gland, making these branches difficult to visualize with conventional US. Wegscheider et al. recently demonstrated the ability to map the facial nerve from the stylomastoid foramen to distal muscle branches using HFUS[34]. This proof-of-concept study shows promise for assessing facial nerve pathology. In a prospective controlled clinical study, Lo et al. demonstrated US can be used to help predict facial palsy outcomes in patients with Bell's palsy[35]. These findings were subsequently confirmed by Baek et al. in 2020 [Figure 3][36].
Figure 3. (A) The ultrasound probe is applied to the target imaging area (A). (B) The facial nerve was identified within the parotid gland. With permission from Baek et al.[36].
Functional US (fUS) is an emerging neuroimaging modality that can visualize and quantify perfusion of nervous structures, with spatial and temporal resolutions of 200 uM and 20 ms reported, respectively[37,38]. This offers highly localized and specific vascular perfusion information, and confers advantages over functional MRI (fMRI), including portability, machine size, and acquisition time. In a pre-clinical study by Réaux-Le-Goazigo et al., an innovative localization and functional ultrasound technique enabled real-time, dynamic functional imaging of the rat trigeminal nerve following nociceptive stimulation[39]. This type of functional ultrasonography utilizes HFUS or UHFUS and applies digital signal processing that resolves temporospatial data to show vascular changes in target tissues. A recent study by Matsui et al. demonstrated the ability to collect detailed quantitative data on intraneural microvascular flow of the ulnar nerve[40]. Using a 14-MHz linear probe and an intravenous microbubble contrast agent, real-time dynamic assessment of intraneural flow was captured[41]. While it does not provide direct information on nerve function itself, nerve perfusion is an important indicator of nerve health[42].
CONCLUSION
Determining the prognosis for traumatic facial nerve injuries can be challenging, and requires accurate and prompt injury assessment for surgical planning. Imaging advancements over the last decade can now generate detailed, high-resolution imaging of the facial nerve and other peripheral cranial nerves, unveiling previously unparalleled detail and morphology.
Innovations in MRI and US capabilities, including DWI and DTI with tractography and high-resolution US, offer promise for refined facial nerve injury assessment, improved prognostication, and ultimately surgical planning. More recently, functional ultrasonography represents an exciting area of innovation that clinicians and surgeons may find useful for assessing and tracking facial nerve injury. Additional prospective studies dedicated to traumatic facial nerve injuries using these modalities are needed.
DECLARATIONS
Author’s contributionsInitial Literature Search: Fung E, Bradley E
Additional Literature Search: Hatala A, Melnyk B, Datta N
Initial manuscript draft: Fung E, Hatala A, Melnyk B, Bradley E
Substantial contributions to the conception and design of the study and performed data analysis, interpretation, and synthesis: Datta N
Significant guidance and feedback in the manuscript editing process and completion of final draft: Datta N
Availability of data and materialsNot applicable.
Financial support and sponsorshipNone.
Conflicts of interestAll authors declared that there are no conflicts of interest.
Ethical approval and consent to participateNot applicable.
Consent for publicationNot applicable.
Copyright© The Author(s) 2023.
REFERENCES
1. Chhabra A, Ahlawat S, Belzberg A, Andreseik G. Peripheral nerve injury grading simplified on MR neurography: as referenced to Seddon and Sunderland classifications. Indian J Radiol Imaging 2014;24:217-24.
3. Gordon T. Chapter 61 - The biology, limits, and promotion of peripheral nerve regeneration in rats and humans. Nerves and Nerve Injuries 2015;2:993-1019.
4. Fu SY, Gordon T. Contributing factors to poor functional recovery after delayed nerve repair: prolonged axotomy. J Neurosci 1995;15:3876-85.
5. Fu SY, Gordon T. Contributing factors to poor functional recovery after delayed nerve repair: prolonged denervation. J Neurosci 1995;15:3886-95.
6. Furey MJ, Midha R, Xu QG, Belkas J, Gordon T. Prolonged target deprivation reduces the capacity of injured motoneurons to regenerate. Neurosurgery 2007;60:723-32; discussion 732-3.
7. Kannan RY, Hills A, Shelley MJ, et al. Immediate compared with late repair of extracranial branches of the facial nerve: a comparative study. Br J Oral Maxillofac Surg 2020;58:163-9.
8. Ein L, Xiao R, Zhou AS, Hadlock TA. Facial nerve exploration for known or suspected transection: the massachusetts eye and ear experience. Facial Plast Surg Aesthet Med 2023;25:306-11.
9. Seneviratne SO, Patel BC. Facial nerve anatomy and clinical applications. Treasure Island (FL): StatPearls Publishing; 2022.
10. Hohman MH, Hadlock TA. Etiology, diagnosis, and management of facial palsy: 2000 patients at a facial nerve center. Laryngoscope 2014;124:E283-93.
11. Tzermpos FH, Cocos A, Kleftogiannis M, Zarakas M, Iatrou I. Transient delayed facial nerve palsy after inferior alveolar nerve block anesthesia. Anesth Prog 2012;59:22-7.
12. Cumming B, Matchett I, Meller C, Saxby A. High altitude alternobaric facial palsy: case series and systematic review of the literature. Otol Neurotol 2019;40:1378-85.
13. Patel A, Groppo E. Management of temporal bone trauma. Craniomaxillofac Trauma Reconstr 2010;3:105-13.
14. Mu X, Quan Y, Shao J, Li J, Wang H, Gong R. Enlarged geniculate ganglion fossa: CT sign of facial nerve canal fracture. Acad Radiol 2012;19:971-6.
15. Gupta S, Mends F, Hagiwara M, Fatterpekar G, Roehm PC. Imaging the facial nerve: a contemporary review. Radiol Res Pract 2013;2013:248039.
16. Chhabda S, Leger DS, Lingam RK. Imaging the facial nerve: a contemporary review of anatomy and pathology. Eur J Radiol 2020;126:108920.
17. Yan C, He B, Wang X, Qin Z, Li Y, Peng Y. Accuracy of high-resolution computed tomography in revealing fallopian canal fracture of patients with traumatic facial paralysis. Auris Nasus Larynx 2015;42:374-6.
18. Chen Y, Zhang K, Xu Y, Che Y, Guan L, Li Y. Reliability of temporal bone high-resolution CT in patients with facial paralysis in temporal bone fracture. Am J Otolaryngol 2018;39:150-2.
19. de Tribolet N. Binder DK, Sonne DC, Fischbein NJ, cranial nerves: anatomy, pathology, imaging. Acta Neurochir 2012;154:1323.
20. Mahale A, Choudhary S, Ullal S, Fernandes M, Prabhu S. Postcontrast fluid-attenuated inversion recovery (FLAIR) sequence MR imaging in detecting intracranial pathology. Radiol Res Pract 2020;2020:8853597.
21. Chu J, Zhou Z, Hong G, et al. High-resolution MRI of the intraparotid facial nerve based on a microsurface coil and a 3D reversed fast imaging with steady-state precession DWI sequence at 3T. AJNR Am J Neuroradiol 2013;34:1643-8.
22. Taoka T, Hirabayashi H, Nakagawa H, et al. Displacement of the facial nerve course by vestibular schwannoma: preoperative visualization using diffusion tensor tractography. J Magn Reson Imaging 2006;24:1005-10.
23. Savardekar AR, Patra DP, Thakur JD, et al. Preoperative diffusion tensor imaging-fiber tracking for facial nerve identification in vestibular schwannoma: a systematic review on its evolution and current status with a pooled data analysis of surgical concordance rates. Neurosurg Focus 2018;44:E5.
24. Li H, Wang L, Hao S, et al. Identification of the facial nerve in relation to vestibular schwannoma using preoperative diffusion tensor tractography and intraoperative tractography-integrated neuronavigation system. World Neurosurg 2017;107:669-77.
25. Szmuda T, Słoniewski P, Ali S, et al. Reliability of diffusion tensor tractography of facial nerve in cerebello-pontine angle tumours. Neurol Neurochir Pol 2020;54:73-82.
26. Jang SH, Seo JP, Kwon YH. Traumatic trigeminal neuropathy after whiplash injury: A case report. Medicine 2022;101:e29012.
27. Epprecht L, Kozin ED, Piccirelli M, et al. Super-resolution diffusion tensor imaging for delineating the facial nerve in patients with vestibular schwannoma. J Neurol Surg B Skull Base 2019;80:648-54.
28. Izzetti R, Vitali S, Aringhieri G, et al. Ultra-high frequency ultrasound, a promising diagnostic technique: review of the literature and single-center experience. Can Assoc Radiol J 2021;72:418-31.
29. Aringhieri G, Izzetti R, Vitali S, et al. Ultra-high frequency ultrasound (UHFUS) applications in Sjogren syndrome: narrative review and current concepts. Gland Surg 2020;9:2248-59.
30. Thotakura AK, Marabathina NR, Danaboyina AR, Mareddy RR. Role of serial ultrasonic optic nerve sheath diameter monitoring in head injury. Neurochirurgie 2017;63:444-8.
31. Newman WD, Hollman AS, Dutton GN, Carachi R. Measurement of optic nerve sheath diameter by ultrasound: a means of detecting acute raised intracranial pressure in hydrocephalus. Br J Ophthalmol 2002;86:1109-13.
32. Rajajee V, Vanaman M, Fletcher JJ, Jacobs TL. Optic nerve ultrasound for the detection of raised intracranial pressure. Neurocrit Care 2011;15:506-15.
33. Helmke K, Hansen HC. Fundamentals of transorbital sonographic evaluation of optic nerve sheath expansion under intracranial hypertension. I. Experimental study. Pediatr Radiol 1996;26:701-5.
34. Wegscheider H, Volk GF, Guntinas-Lichius O, Moriggl B. High-resolution ultrasonography of the normal extratemporal facial nerve. Eur Arch Otorhinolaryngol 2018;275:293-9.
35. Lo YL, Fook-Chong S, Leoh TH, et al. High-resolution ultrasound in the evaluation and prognosis of Bell's palsy. Eur J Neurol 2010;17:885-9.
36. Baek SH, Kim YH, Kwon YJ, et al. The utility of facial nerve ultrasonography in bell's palsy. Otolaryngol Head Neck Surg 2020;162:186-92.
37. Soloukey S, Vincent AJPE, Satoer DD, et al. Functional ultrasound (fUS) during awake brain surgery: the clinical potential of intra-operative functional and vascular brain mapping. Front Neurosci 2019;13:1384.
38. Demene C, Baranger J, Bernal M, et al. Functional ultrasound imaging of brain activity in human newborns. Sci Transl Med 2017;9:eaah6756.
39. Réaux-Le-Goazigo A, Beliard B, Delay L, et al. Ultrasound localization microscopy and functional ultrasound imaging reveal atypical features of the trigeminal ganglion vasculature. Commun Biol 2022;5:330.
40. Matsui Y, Horie T, Funakoshi T, Kawamura D, Nishida M, Iwasaki N. Dynamic evaluation of intraneural microvascularity of the ulnar nerve using contrast-enhanced ultrasonography in patients with cubital tunnel syndrome. J Hand Surg Am 2022;47:687.e1-8.
41. Ishizaka K, Nishida M, Motomiya M, et al. Reliability of peripheral intraneural microhemodynamics evaluation by using contrast-enhanced ultrasonography. J Med Ultrason 2014;41:481-6.
Cite This Article
Export citation file: BibTeX | RIS
OAE Style
Datta N, Fung E, Hatala A, Melnyk B, Bradley E. Imaging traumatic facial nerve injuries: a narrative review of current strategies and future directions for cranial nerve imaging. Plast Aesthet Res 2023;10:51. http://dx.doi.org/10.20517/2347-9264.2023.22
AMA Style
Datta N, Fung E, Hatala A, Melnyk B, Bradley E. Imaging traumatic facial nerve injuries: a narrative review of current strategies and future directions for cranial nerve imaging. Plastic and Aesthetic Research. 2023; 10: 51. http://dx.doi.org/10.20517/2347-9264.2023.22
Chicago/Turabian Style
Datta, Néha, Ethan Fung, Adam Hatala, Brooks Melnyk, Erika Bradley. 2023. "Imaging traumatic facial nerve injuries: a narrative review of current strategies and future directions for cranial nerve imaging" Plastic and Aesthetic Research. 10: 51. http://dx.doi.org/10.20517/2347-9264.2023.22
ACS Style
Datta, N.; Fung E.; Hatala A.; Melnyk B.; Bradley E. Imaging traumatic facial nerve injuries: a narrative review of current strategies and future directions for cranial nerve imaging. Plast. Aesthet. Res. 2023, 10, 51. http://dx.doi.org/10.20517/2347-9264.2023.22
About This Article
Copyright
Data & Comments
Data
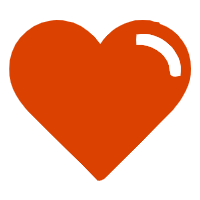

Comments
Comments must be written in English. Spam, offensive content, impersonation, and private information will not be permitted. If any comment is reported and identified as inappropriate content by OAE staff, the comment will be removed without notice. If you have any queries or need any help, please contact us at support@oaepublish.com.